Scientists from IISc are addressing questions about the biological world using their engineering skills
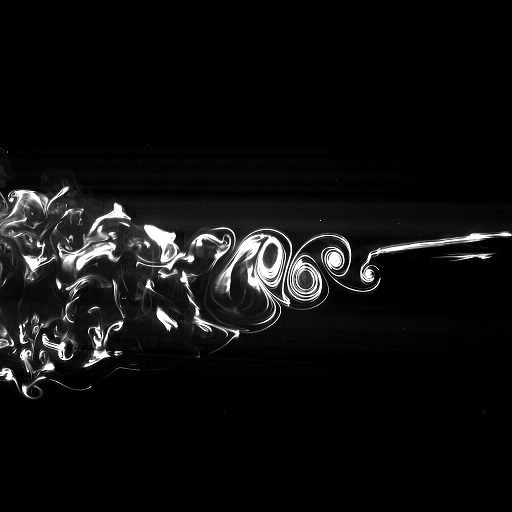
Most mechanical engineers do what we think they do – design and fabricate aircraft, machines, and other such human-made objects. But it turns out that some members of their tribe are also curious about how living things work.
One of them is Namrata Gundiah, Professor in the Department of Mechanical Engineering, who has taken on the challenge of addressing problems in biology using her training in mechanics.
Elastin and collagen
When Namrata began her PhD at the University of California, Berkeley, she was drawn to a class of rubber-like proteins secreted by cells in certain tissues that allow them to undergo large and cyclic deformations. Elastin is one such protein responsible for the “stretchiness” of tissues.
This led Namrata to investigate what happens to elastic tissues in the human cardiovascular system – specifically arteries and the heart – when they are damaged during disease. “When I, in collaboration with a cardiac surgeon, saw how damaged tissues adapt following injury, I was fascinated with how the cellular processes could contribute to changes in the tissue properties. Exploring changes to tissue properties after disease then became the subject for my postdoc for a two-year period.”
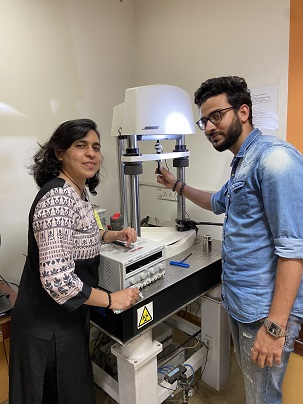
Anshul Shrivastava studying hydrogels (Picture courtesy: Namrata Gundiah Lab)
Namrata decided that if she were to study how tissue properties change during disease (and how to design engineering solutions to handle disease), then she would have to use a “mechanobiology approach.” This emerging field of science, which brings together physics, engineering and biology, attempts to understand how mechanical properties affect the fates of cells and development of tissues. Today, mechanobiology has become an integral part of her research. The questions Namrata’s lab is addressing in the realm of cell-based mechanics are how cells adhere to the substrate, how they respond to mechanical cues, and how they migrate.
Another important focus of research for Namrata is aortic aneurysm, in which the walls of the aorta, the largest blood vessel in the body, become weak and bulge when blood flows under high pressure. This may cause the aorta to rupture. One of the primary drivers of this disease is the disruption in the balance between collagen, the main structural protein found in human beings and other mammals, and elastin, the rubber-like protein that she had studied earlier. “There’s an imbalance between the two proteins – not having enough elastin and the deposition of collagen of poor quality,” she says. She and her team created a disease model by changing the relative composition of the two proteins, and quantified the mechanical properties of the tissues. The ideas were tested using human aortic samples obtained from Narayana Hrudayalaya (now Narayana Health City) in Bangalore.
Hydrogels
Namrata also uses tissue engineering, a discipline that aims to restore or replace damaged tissues in the human body. Here, scaffolds of a specific material are engineered, and tissues are grown on them in a laboratory. Among the more popular tissue engineering scaffolds are hydrogels. Hydrogels are made up of large molecules or polymers that have a unique combination of properties: they do not dissolve in water, are absorbent, and are structurally sound.
In the future, hydrogels could have significant applications as biomaterials. For instance, the cornea, which is the clear, protective outer layer of the eye, is similar to a hydrogel. Like many other parts of the human body, the cornea contains the protein collagen. When collagen degrades, it damages the cornea. Namrata’s lab is interested in synthesising hydrogels that are tougher so that they can be used to help corneal wounds heal. “If we are looking to the next generation of biomaterials, we need materials that are tough, can support cell growth and can serve as replacements for tissues,” she explains.
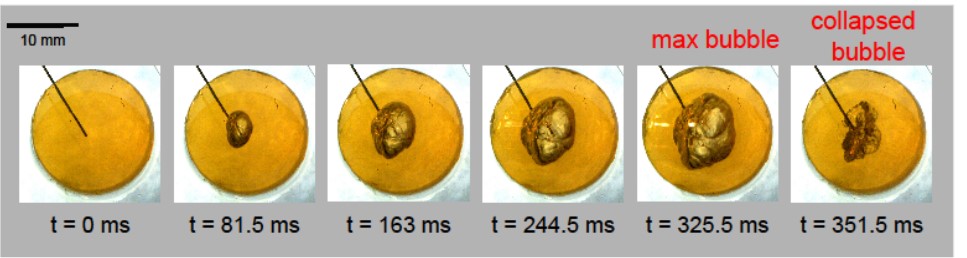
Another important reason why bioengineers are interested in hydrogels is the way they rupture. “They can undergo large deformations and when they fail, they are brittle and rupture not unlike jelly, the dessert made from gelatin. Jelly has a lot of water, and can undergo large deformations, but when you break it, it has a very clean rupture surface. It breaks like glass,” Namrata explains. She says that understanding the mechanics behind hydrogel failure may provide insights into tissue engineering applications.
Insect biology
When Namrata decided to set up her lab at IISc, she remembered what one of her mentors from her graduate school had told her: “When you set up your lab, you should have your bread-butter projects which will give you the moolah for your research. But always have a hobby project which you do purely for the joy of science.” She took his advice seriously and decided that her hobby project was going to be insect biomechanics. She began with resilin, one of the best-known rubber-like proteins, found in the appendages of insects and other arthropods.
Namrata’s insect biology research has thrown up many surprises. For instance, her lab has shown that the female parasitic fig wasp inserts her ovipositor (the appendage used for egg-laying) inside the fruit with the help of a microscopic drill enriched with zinc. Her team has also shown that mandibles of the larvae of the coffee white stem borer beetles are strengthened by zinc too. They use their mandibles to tunnel their way through wood until they find a safe place to pupate. “My student wanted to call it “cutting-edge” research, while I call it “boring” research,” she says in jest.
Swimming fish
Jaywant H Arakeri, Professor, and Namrata’s colleague at the Department of Mechanical Engineering, is also an engineer who has a passion for the biological world. One of the problems that this fluid mechanist studies, along with RN Govardhan, also a Professor in the Department, is how fish and other aquatic animals glide through water.
When an object moves forward underwater at high speeds, the forward forces, or thrust, need to balance the forces that push it back. These forces, called drag, are the result of friction and pressure exerted by water on the moving body.
“If you look at a submarine, it has a propeller whose rotation helps it overcome the drag on it. The blades of propellers are rigid. But if you look at a fish, it’s body and tail are flexible,” says Jaywant. The other difference between a human-made propeller and the tail of a swimming fish is the unsteady nature of movement in the latter. “Unlike a propeller which moves at a constant speed, the fish tail constantly moves back and forth, with its speed periodically varying from zero to a maximum value.” Jaywant’s lab is studying how these factors – the flexibility of the body and tail of the fish and the flapping of its tail – contribute to both thrust and drag.
Jaywant’s lab is studying how these factors – the flexibility of the body and tail of the fish and the flapping of its tail – contribute to the forces of thrust and drag
Jaywant’s team is also addressing another related question: how efficiently do fish swim when compared to human-made objects? “There is no clear definition of efficiency. But one way of thinking about it is how much energy is expended,” he says. The energy expended shows up as disturbance in the wake, the region of disturbed flow of water around a body, which can be measured. “Many people believe that the wake of a fish is quieter than that of human-made objects.”
Jaywant’s experiments are done in a water tunnel or in a glass tank containing water. An airfoil having a flexible flap is connected to a motor that produces oscillatory motion. “This makes it behave like a fish and we are able to measure the forces it experiences. It tells us about the thrust being generated. We can also measure the torque [rotational force],” he explains. The torque reveals how efficiently the thrust is generated.
And in order to visualise flow, a fluorescein dye is used. By shining a sheet of laser light perpendicular to the foil, researchers can see the wakes and vortices (a vortex is a whirling mass of water produced by the movement of the swimming object) that are formed. His team also makes quantitative measurements of the velocity around a “swimming” foil. “For this, we use a technique called particle image velocimetry where you put really minute tracer particles of the order of 10-20 microns in water and shine a laser. And with a high-speed camera, we can measure the displacement of these particles,” he elaborates.
Agriculture
Jaywant comes from a family of agriculturists. “My father was an agricultural scientist, and many of my family members are into farming. So it was natural that we started looking at fluid mechanics issues related to agriculture,” he says.
The first agricultural problem that Jaywant tackled was how temperature could be regulated in a polyhouse, a kind of a greenhouse protected by a polythene mesh. Polyhouses, commonly used for growing vegetables and flowers, are of two kinds: one with a fan pad system which reduces temperature by evaporation of water constantly dripping from a wet pad. The other is a naturally-ventilated polyhouse, in which temperatures can go up substantially, especially in a tropical country like India.
To make polyhouses more energy-efficient, Jaywant has collaborated with MS Bobji (Professor, Department of Mechanical Engineering), M Uday Kumar (Professor, University of Agricultural Sciences, Bangalore), and KR Sreenivas (Professor, Jawaharlal Nehru Centre for Advanced Scientific Research) on projects funded by the Robert Bosch Centre for Cyber Physical Systems at IISc and the Indian Council of Agricultural Research. As part of one of the projects, they developed a design for a naturally-ventilated polyhouse that incorporates a solar chimney. The chimney uses the convection of air heated by solar energy. “We wanted to see if evaporation could be built into the system, while also being energy-efficient.”
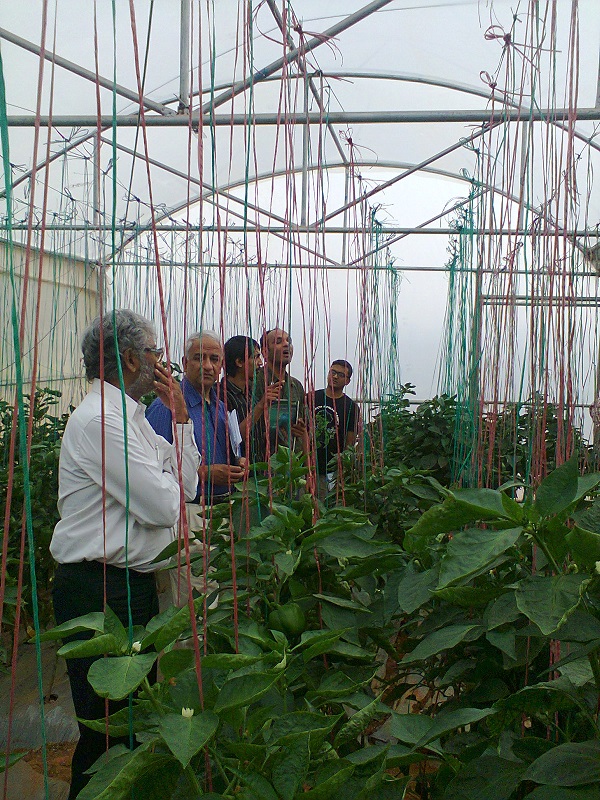
Yet another agricultural project was to create optimal conditions in aeroponics chambers used to grow potato tubers for seed production. Aeroponics is a method of cultivation where the roots, hanging in the air, absorb moisture and nutrients from tiny droplets that are continually sprayed on to them. “We came up with cost effective and energy efficient techniques to keep temperatures low, at both the root and shoot levels, by using fluid mechanics and heat transfer principles in the design,” says Jaywant. His team has also investigated how supplemental LED lighting can be used to increase tuber production.
Jaywant’s research interests in agriculture do not end there. “My students and I have come up with a small device to rapidly measure evaporation rates at the leaf level.” This, he says, will tell us how much water is required by plants – and when – as we move towards precision agriculture.
Jaywant has also collaborated with Namrata to study arterial flows to understand flow-induced stresses that are believed to be responsible for several cardiovascular diseases, including atherosclerosis.
Jaywant’s and Namrata’s curiosities about living organisms have inspired them to make intellectually exciting journeys from the world humans have built to the biological world. And in the process, the mechanical engineers have provided us with a unique perspective of nature.
For more stories on the Department of Mechanical Engineering, please click on the links below:
Making Things Work: 75 Years of Mechanical Engineering
Remembering Arcot Ramachandran
Out of the Lab, Into the World Beyond
Building a Testament to ME History