The lensing of waves is helping us look deeper into space
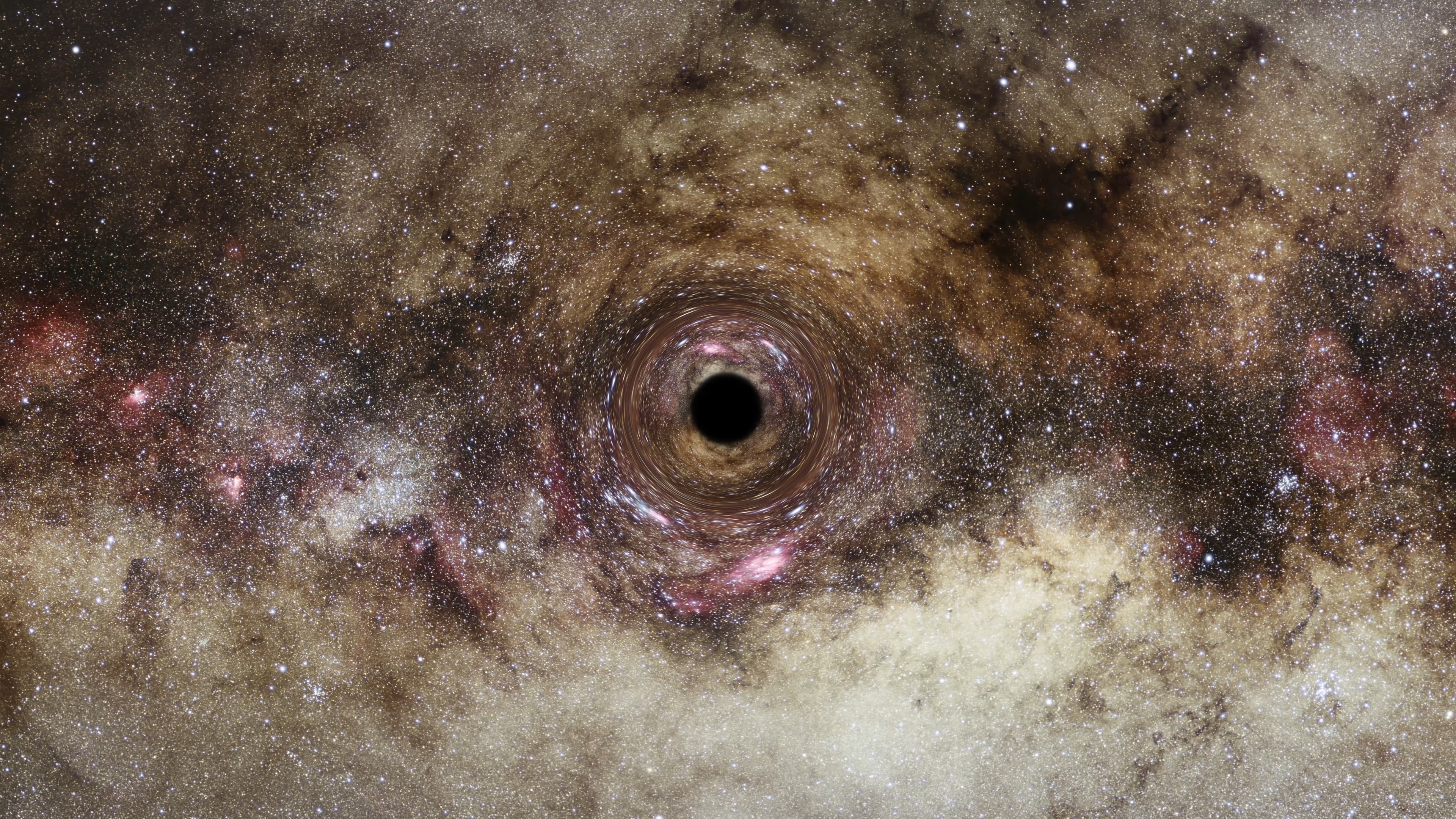
Arthur Eddington was growing anxious. It was nearly 2 pm on 29 May 1919. The British astronomer was standing on a plateau overlooking the Atlantic Ocean in the west African island of Príncipe. A rare solar eclipse, not seen in 500 years, was about to begin. It should have been a hot and clear day. But thunder was rolling across the overcast sky, threatening to ruin his plans.
Some years earlier, a friend had written to Eddington about a young scientist named Albert Einstein who had just published his theory of general relativity, making extraordinary claims about the nature of gravity. With World War I raging, few people outside Germany knew or cared about these ideas, more so because they defied theories proposed by the better-known Isaac Newton. But Eddington was impressed with Einstein’s work and wanted to help him.
One of Einstein’s claims was that the gravitational field around the sun would bend light rays coming from a distant object as they skirted the sun’s rim. Eddington and Frank Dyson, Britain’s Astronomer Royal, came up with a clever way to prove this. They would first take photos of a bright star cluster called the Hyades close to the sun, which was only possible when the sun was blacked out during a total eclipse. They would then cross-check this with the Hyades’ position a few months later when the sun had moved away. If the sun bent the stars’ light, their position during the eclipse should appear off by an angle of 1.75 arcseconds (about 1/60th of a millimetre on a photographic plate), according to Einstein’s calculations. Two locations where the eclipse would be clearly visible were chosen: Príncipe – where Eddington and technician Edwin Cottingham set up camp – and Sobral, a city in Brazil where astronomers Andrew Crommelin and Charles Davidson were dispatched.
At 1.55 pm, miraculously, the clouds cleared over Príncipe. Five seconds past 2.13 pm, the eclipse’s totality began, and the team managed to take 16 still photographs during the five minutes that the eclipse lasted. When they completed their calculations a few months later, the Príncipe team found that the star positions were off by about 1.61 arcseconds, while the Sobral data suggested around 1.98. On 6 November 1919, at a joint meeting of the Royal Society and Royal Astronomical Society in London, the researchers announced their findings, turning Einstein into a star overnight.
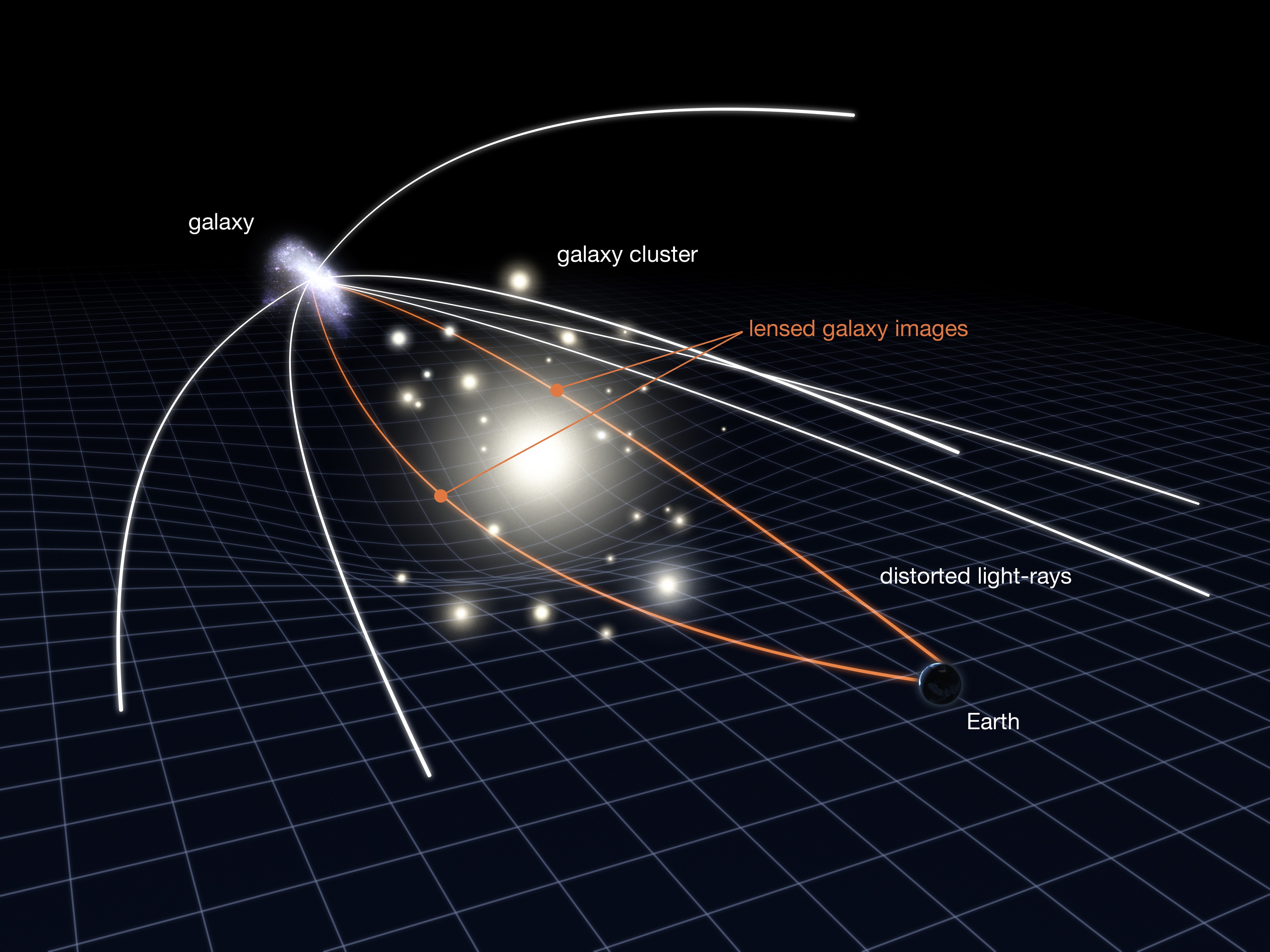
The eclipse experiment was not just a validation of Einstein’s ideas. It was also the first demonstration of a phenomenon called gravitational lensing.
The universe is made up of billions of stars, planets, and galaxies. But many of these are either too faint or too far away for our telescopes to spot. Sometimes, by a stroke of luck, light rays from one of these objects encounter a massive body – like a galaxy cluster – en route to Earth. This body can act like a gigantic lens, curving the light rays around itself and magnifying their intensity. Instead of a pinprick of light or no light at all, what our telescopes might sometimes see are bright banana-like arcs of light surrounding the image of the lensing body in the centre. Each light ray can reach the Earth at different times, resulting in a time delay between different arcs corresponding to the same original object. Not just visible light – other parts of the electromagnetic spectrum like radio waves and gamma rays can also be lensed and magnified.
Gravitational lensing has helped astronomers pinpoint hundreds of new objects in the sky, map the distribution of matter across space, and even probe the origins of our universe.
Gravitational lensing has helped astronomers pinpoint hundreds of new objects in the sky, map the distribution of matter across space, and even probe the origins of our universe
“It helps you study both the object that’s being lensed and the one doing the lensing,” explains Nirupam Roy, Associate Professor at the Department of Physics, IISc. For example, how much the light is bent can tell us how big the lensing body is – if it is massive, the bending can be extremely strong. Take Gargantua, the fictional supermassive black hole featured in the movie Interstellar. Streams of light are bent so strongly by it that they loop and curve around it several times, giving it an ethereal look. “This is effectively gravitational lensing,” Nirupam says.
Gases in galaxies
It was strong gravitational lensing that helped Nirupam and his collaborator Arnab Chakraborty detect a radio signal from a galaxy billions of light-years away from Earth.
A few years ago, while poring over images captured by the Hubble Space Telescope, Nirupam and Arnab chanced upon a far-off galaxy. Its light had been lensed so strongly by an intervening galaxy cluster that instead of arcs, it created a bright, nearly full circle of light, called an Einstein ring.
What the duo was more interested in, however, was the galaxy’s gas composition, particularly atomic hydrogen gas, which is the fuel for star formation. But atomic hydrogen does not emit visible light. The only way to detect it is to look for a 21 cm spectral line – a radio wave of a specific wavelength generated when there is a change in the energy state of the atoms. To search for this line, the researchers used the Giant Metrewave Radio Telescope (GMRT) – an array of large parabolic antennas – near Pune, Maharashtra.
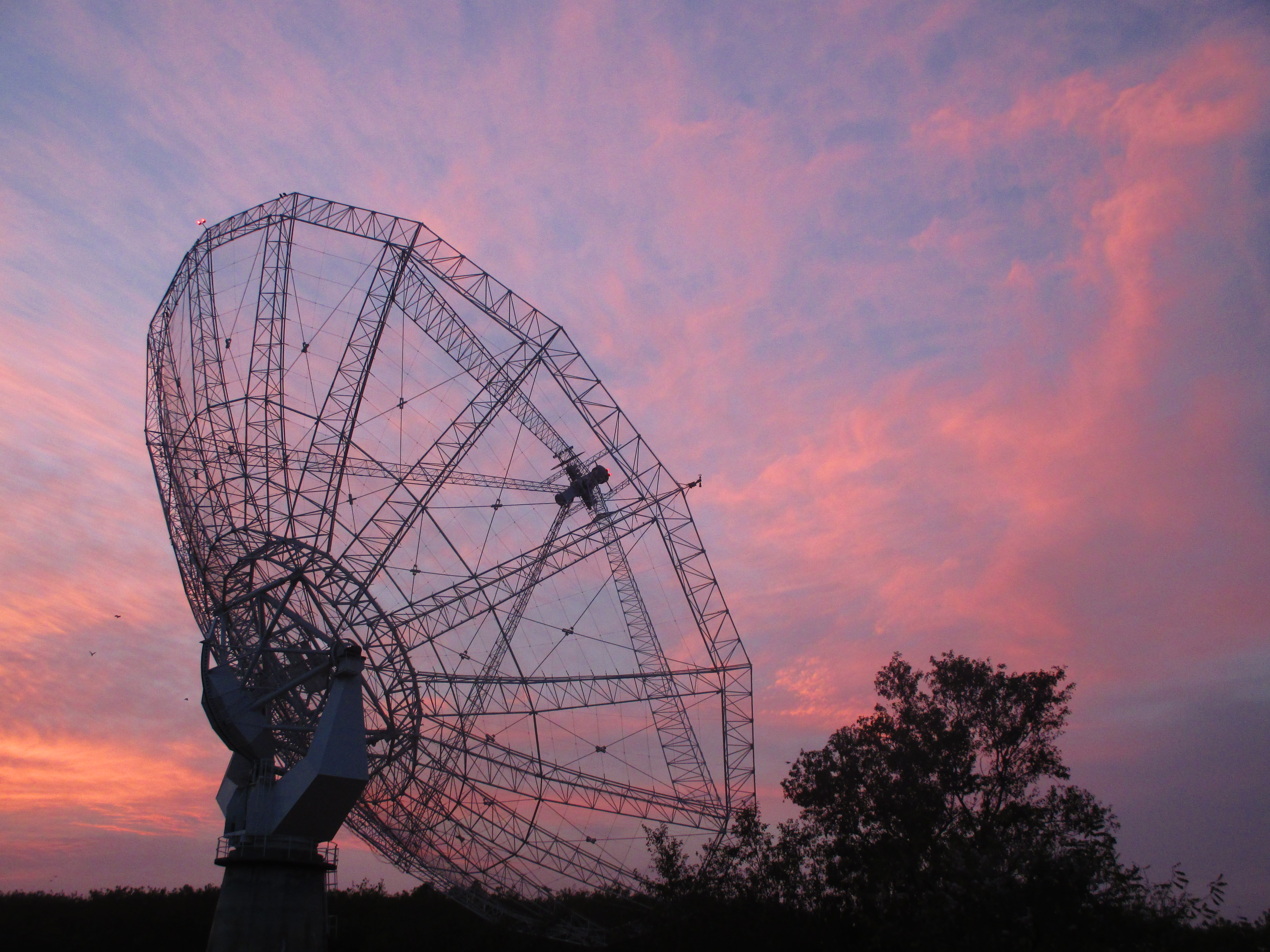
Detecting such radio waves is challenging, because they are weak compared to star light, and can also be disrupted by radio signals from earthly sources like cell phones. Normally, there was no way that even GMRT, among the most sensitive radio telescopes on Earth, could have picked up the signal.
Luckily, the same cluster that lensed the galaxy’s light also ended up lensing its 21 cm line, magnifying the latter by nearly 30 times, allowing GMRT to pick it up. “[Otherwise], it would have taken us, say, 900 times longer observation time, or we would have had to build a telescope with about 30 times larger collecting area,” explains Nirupam. It was and remains the farthest distance from which such a signal had been picked up. It was also the first time anybody had detected a strongly lensed 21 cm line from any galaxy.
Analysing the lensed radio signal gave the team several new insights. They found that it had originated about eight billion years ago, but the galaxy was so far away that we were only now able to detect it. “You are [essentially] looking back into the past,” says Nirupam. They were also able to work out that the atomic hydrogen mass in the galaxy at that time was twice as high as the mass of its stars – higher than one would expect to see in galaxies now. This led them to speculate that early-stage galaxies were probably more gas-rich because a lot more stars were forming in them.
Analysing the lensed radio signal gave them several insights. They found that it had originated eight billion years ago
“We are now planning to put in requests for observation time at different telescopes so that we can try to detect this gas in many more sources,” Nirupam says. “If we can do this for a larger sample, we can make a more useful statement about the property of galaxies in general in the distant universe.”
Ripples in spacetime
One hundred years after Einstein’s theory of general relativity was published, on 14 September 2015, scientists gathered at twin facilities in the USA that together make up the Laser Interferometer Gravitational-Wave Observatory (LIGO), waiting anxiously for results that could prove another of his predictions.
Einstein had suggested that gravity arises when massive objects stretch the fabric of spacetime, like a bowling ball on a trampoline. During violent events, like two black holes smashing into each other, the stretching is so strong that it creates tiny, fast-moving ripples in this fabric called gravitational waves. These waves are not part of the electromagnetic spectrum, are extremely weak, and cannot be “seen”.
To catch these waves, scientists at LIGO built two 4 km-long perpendicular vacuum tubes or “arms” with mirrors at each end. A laser beam split into two travels across each arm, keeping track of the distance between the mirrors. If a gravitational wave passes through, it would stretch the space inside the arm ever so slightly, changing the light’s path length by an incredibly tiny amount – about 10-19 metres. It was this change that the team caught that day for the first time – it showed up as a ‘chirp’ or peak in the signal captured by LIGO’s detectors. By analysing the chirp, they deduced that the waves had come from the collision of two black holes 1.3 billion years ago, which created a burst of gravitational waves in the final milliseconds.
Since then, about 100 gravitational wave signals have been detected, unveiling objects and events hidden from optical and radio telescopes. “For example, gravitational waves are probably the only way of detecting a double black hole merger because it doesn’t emit any light,” explains Parameswaran Ajith, Professor at the International Centre for Theoretical Physics (ICTS), Bangalore, who is part of the LIGO collaboration. “In the last eight years, observations of gravitational waves have told us that these collisions of black holes are rather ubiquitous in the universe.” Studying gravitational waves can also tell us more about the universe’s ancient past. “The earliest we can ‘see’ using electromagnetic waves is … about 300,000 years after the Big Bang,” Ajith adds. “We cannot ‘see’ the universe beyond that because the universe was so dense that light could not travel. But gravitational waves could.”
Studying gravitational waves can tell us more about the universe’s ancient past
Crucially, gravitational waves can also be lensed, in theory. Magnified, repeated chirps echoing the same event can potentially appear at different times in the gravitational wave data. Ajith’s research focuses on devising ways to find these chirps. “Our group has developed the first method to search for gravitational lensing of gravitational waves using a Bayesian statistical model,” he says. The method provides a way to determine if repeated signals popping up in the LIGO data belong to the same event – which means that lensing is happening – or to two different events.
In another study, Ajith and others speculated that the time delay between lensed gravitational waves could be linked to the rate at which the universe is expanding, a value that scientists are yet to agree upon. The team modelled black hole mergers and galaxy clusters as the source and lens respectively. What they found was that if there are more lensed signals or the time delay is smaller than expected, it might point to a larger value of the Hubble constant, which is a measure of the expansion.
However, to validate such predictions, we would need to detect and analyse thousands of such lensed gravitational waves – we are yet to see even one. But scientists are already working on improving current detectors and building new ones, Ajith says. “We are on the verge of a discovery … there is a real possibility of detecting them in the next five years.”
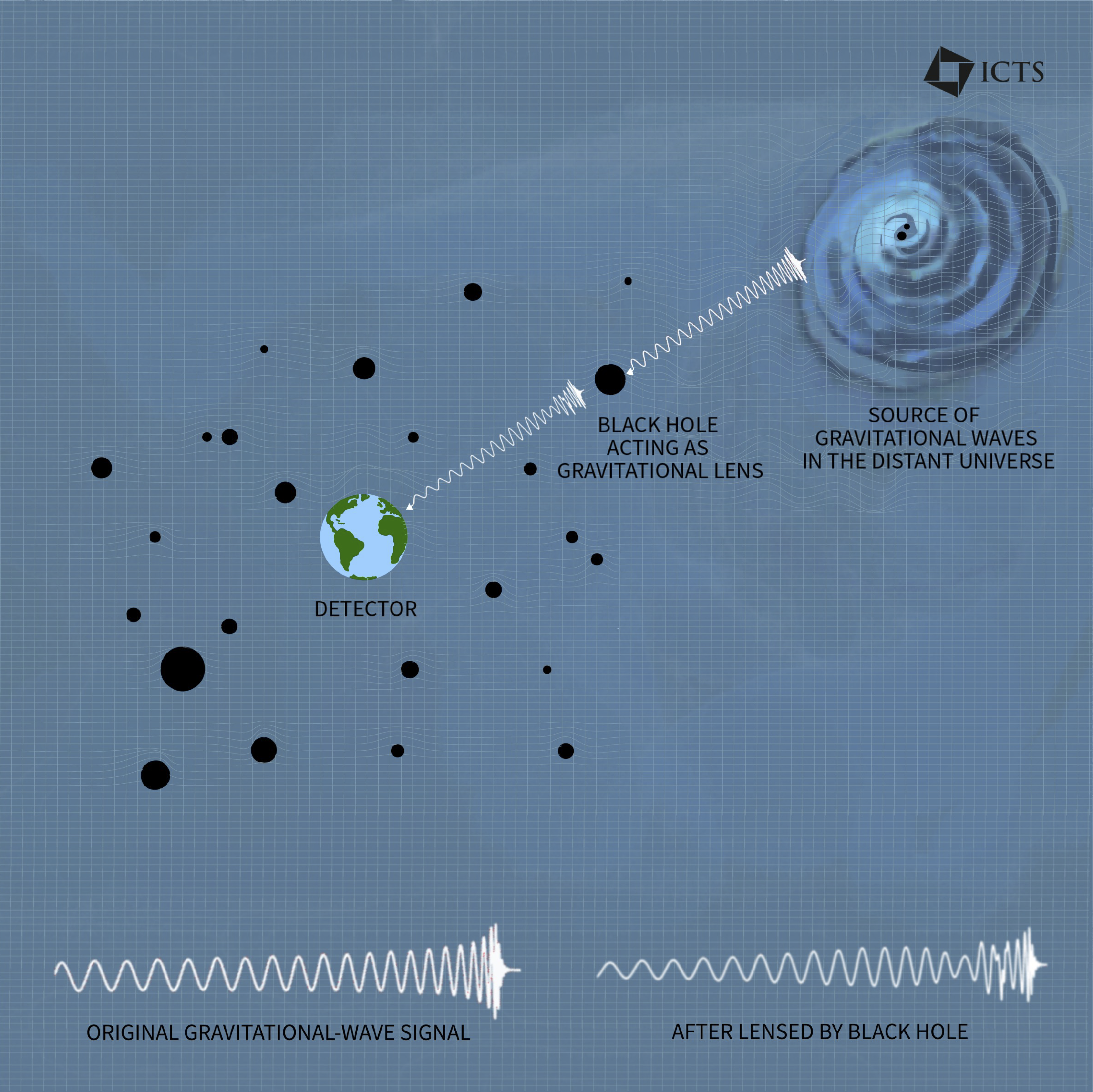
Darkness in the universe
Working late one night in 1963, astronomer Vera Rubin was eating ice cream and mulling over something strange in spectral data collected from the Andromeda galaxy, our closest neighbour. Stars at the edges of the galaxy – which were too far away from the gravitational pull of its core – seemed to be moving at the same speed as stars closer to the centre. She and her collaborator Kent Ford realised that there must be some invisible mass at the galaxy’s fringes providing extra gravity to keep those stars stable. Her work – which many thought should have won a Nobel Prize – made the world wake up to the presence of this invisible mass that we now know as dark matter.
Scientists think that 85% of the universe’s mass is made of dark matter. Although we cannot detect it directly, we know it exists due to its gravitational pull on stars and galaxies. The biggest questions about dark matter are what it is made of, and how it is distributed in and around galaxies. Gravitational lensing is helping scientists answer both.
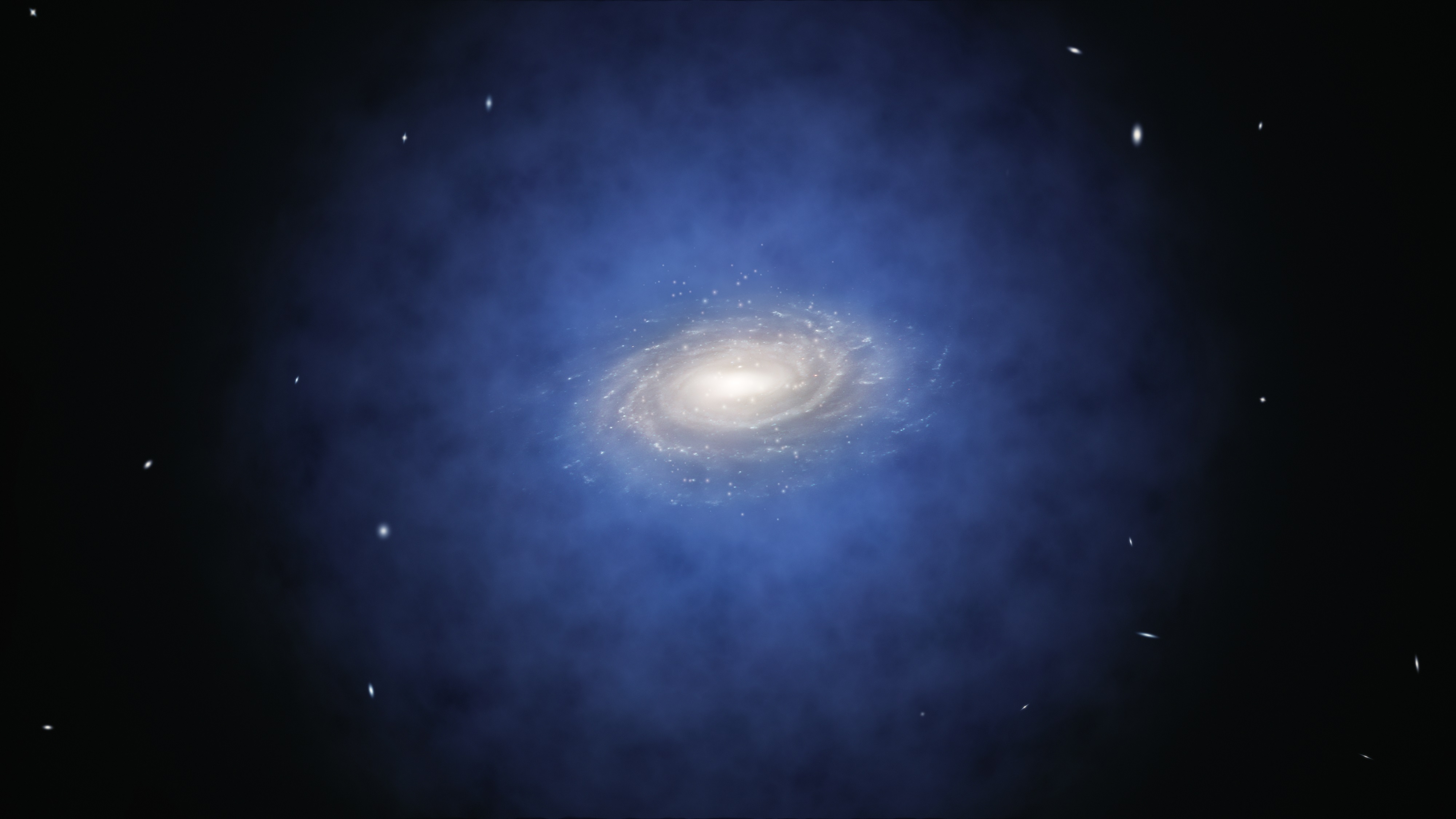
For example, if we calculate the lensing object’s mass based on telescope observations and find that the lensing is much stronger than expected for that mass, the additional bending must be due to the presence of dark matter in the object. Sometimes, dark matter in the space between the Earth and a distant object can subtly bend light rays from the object, creating a smear or distortion instead of bright arcs, a phenomenon called weak lensing. If we compare the shape of the object to the shape of the smear, we can indirectly gauge how much dark matter is present between the Earth and the object.
“We are yet to have a definite answer on what exactly constitutes dark matter,” says Anupreeta More, Research Faculty at the Inter-University Centre for Astronomy and Astrophysics (IUCAA), Pune. “There are various theories. Is it made of some exotic particles? Is it just ordinary matter that is not luminous enough yet?”
The biggest questions about dark matter are what it is made of, and how it is distributed in and around galaxies. Gravitational lensing is helping scientists answer both
A few years ago, Anupreeta was part of an international collaboration that tested one hypothesis: Could dark matter be made of primordial black holes – extremely tiny black holes formed during the earliest seconds after The Big Bang? The researchers used a powerful telescope called Subaru located in Hawaii to first take a full-scale photo of the Andromeda galaxy. If primordial black holes pervaded the galaxy, they would have created many transient lensing events – a phenomenon called microlensing – that would appear as tiny blips. The team expected to see at least 1,000 such blips. Instead, they saw just one. This suggested that primordial black holes probably make up a very tiny fraction of dark matter.
Another theory, which Ajith’s team tested very recently, was whether dark matter could instead be made of much more massive black holes – 100-100,000 times as massive as the Sun. “Our study suggests that because we have not seen any lensed gravitational waves in the last eight years, not more than about 80% of dark matter could be in the form of such massive compact objects,” he explains. “It’s a different way of probing the nature of dark matter.”
Lenses across the sky
On 29 March 1979, astronomers Dennis Walsh, Bob Carswell and Ray Weymann were at the Kitt Peak National Observatory in Arizona, USA, where Rubin had made her groundbreaking observations. Looking through its telescope, they noticed what they thought were two quasars – bright objects found near galaxy centres – close to each other. When they compared the image with spectroscopic signatures, they realised that it was actually two images of the same quasar, lensed by a massive galaxy in between. That was the first official gravitational lens to be spotted. Since then, only a few thousand lenses have been found – a miniscule amount compared to the billions of objects in the universe.
“A lot of my work has been about how to find a large number of lens systems, and how to make sure that we are finding all of them,” Anupreeta says. Her early work focused on developing algorithms to spot lenses in images obtained from large cosmological surveys, but they weren’t efficient enough. In 2013, she and her collaborators launched a citizen science project called Space Warps – these images would be uploaded on a public portal, and anybody could scan them to search for gravitational lenses. “Most of the time, the lensing galaxy tends to be an early-type or elliptical galaxy, and many of these look similar. Our eyes and brains are good at recognising such patterns,” Anupreeta explains. Millions of volunteers from around the world have been helping researchers find new lenses.
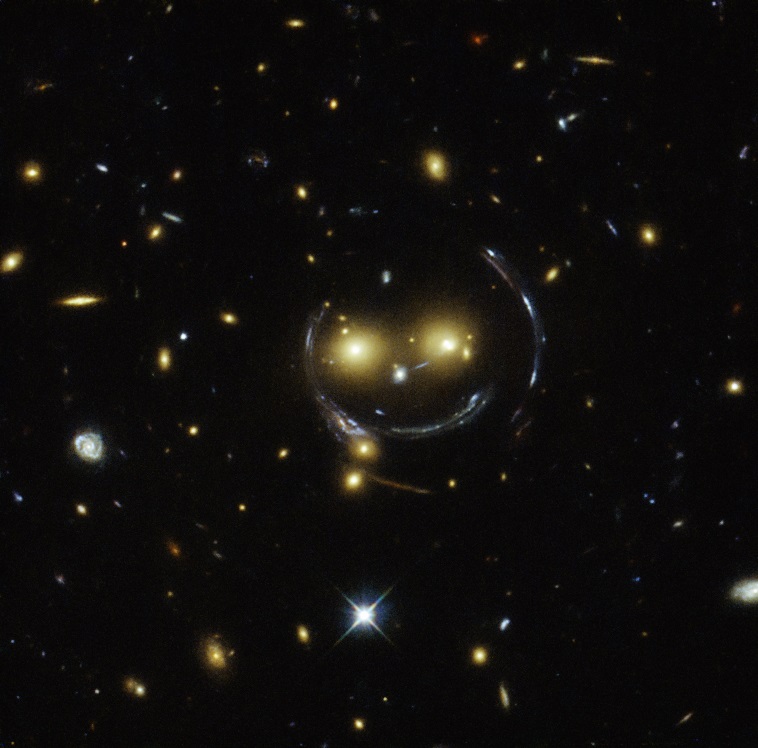
Finding new gravitational lenses will become more significant in the coming years as we enter an era of next-generation telescopes and detectors that will produce staggering amounts of data and images. In Chile, a new observatory named after Rubin will start sweeping skies across the southern hemisphere soon, snapping millions of photos each night. Construction of the Square Kilometre Array – thousands of antennas that will together make up the most sensitive radio telescope in the world – is nearing completion in South Africa and Australia. In India too, a state-of-the-art gravitational wave detector is being built in Hingoli, Maharashtra under the LIGO-India initiative. Dozens of scientists in the country including Ajith, Anupreeta, and Nirupam are involved in such efforts.
“We are very excited about LIGO-India. We haven’t done projects of this scale in fundamental science in India,” Ajith says. “This is a golden opportunity for us to be at the forefront of this research frontier.”