After centuries of sky-watching, we are yet to understand the complex nature of clouds
(Photo: Narmada Khare)
It’s an early winter dawn. The lake seems to be steaming, but it is not. A cloud is being born.
I am on a hilltop, watching a village in the valley sparkling in the early morning rays when a white sheet of thick fog rolls down along the slopes to shroud it completely. This fog is a cloud.
The contrail left behind on the clear blue sky by a passing plane, the mist created when I blow warm moist air from my mouth into the cold, dry morning – those too are clouds.
More spectacular, of course, are the actors in the cosmic drama that we have been witnessing in the skies over Bangalore these last few months. Gossamer white puffs shoved aside in seconds by a roiling army of elephantine greys. Bulbous, discrete clouds merging into a thick, amorphous sheet breached occasionally by crackling branches of lightning. And after the rains, the calming of the skies, disappearing clouds, soft pink brush-strokes, orange swirls and curls left behind, glowing in the setting sun.
Poets and artists have been fascinated by the shapes and colours of clouds, and their movements. So mysterious were they that ancient religions placed gods in charge of controlling clouds. But what about scientists and philosophers? For a phenomenon in nature that is so hard to ignore – clouds cover up to 70% of the globe at any given time – and something that so fundamentally affects our lives, systematic studies of clouds are few and far in between.
“What Jupiter? Do not trifle. There is no Jupiter.”
It isn’t as if civilisations weren’t trying to understand clouds. Aristophanes, the Greek playwright, wrote his play “The Clouds” in the fifth century BCE. The character of Socrates, the philosopher, while denying the need for the thunder god Jupiter to create rain, explains beautifully how water-bearing clouds roll and thunder, burst and clap, and are borne along by vortex. A surprisingly accurate account for its time. About 10 centuries later, the poet Kalidasa, in his Sanskrit play “Meghaduta” (Cloud Messenger), charts the journey of a cloud, rising from the streams, moving north, guided by winds, followed by migrating birds, colliding with cliffs, watched by thirsty fields. This description of the movement of a monsoon cloud is also considered quite accurate. And yet, it was not until much later that scientists like Robert Hooke (17th century) and Jean-Baptiste Lamark (18th century) attempted to actually name different forms of clouds.
Hooke’s suggestions for formally naming the skies – “cleer, hazy, thick, hairy, water’d, Mackeril” and so on – did not take root, but this is considered by some the beginning of the new science of meteorology, as recorded by Humphrey Jennings in his book on contemporary history, Pandaemonium. Eventually, in 1802, Luke Howard, a pharmacist from London, put forth an elegant, universal method of classifying clouds – “water suspended in the atmosphere” – by their appearances, thus putting an end to the notion that their study was “a useless pursuit of shadows”.
Young sun and frozen Earth
Let’s put aside for the moment the cloud-making gods, but there is no denying that many cosmic mysteries need solving before we understand how water started cycling upon our planet – for the water cycle is the main reason why there is life here.
In its early days, Earth may have had all its water either trapped in terrestrial crevices or as hard, icy cliffs. In 1972, noted astronomer Carl Sagan presented a possibility from astrophysical calculations that when the sun was young, it was only 70% as luminous as it is today. Prosenjit Ghosh, Professor at the Centre for Earth Sciences at IISc, who studies paleoclimatology, says, “This faint young sun was not heating the Earth much, and you would expect it to be covered with cold ice. But geologists have found evidence of prehistoric sedimentation along water bodies, which could only happen if there was flowing water.”
The only explanation for what drove this water circulation in spite of the faint sun is the presence of greenhouse gases – CO2 and methane – that warmed up the Earth’s atmosphere like a blanket, thus thawing the ice. “For a billion years after its birth, the Earth was loaded with CO2,” says Prosenjit, “not unlike the atmospheres on Venus and Mars. But unlike those planets, it had plenty of water that evaporated, filling the skies with clouds.”
And what did these clouds do? The same thing that they do today: They both cooled and heated the Earth, depending on how thick they were and at what height. “Low, thick clouds reflect a lot of the Sun’s heat, often cooling the planet,” explains fluid dynamicist Rama Govindarajan, Professor at the International Centre for Theoretical Sciences (ICTS), at a talk given at The Institute of Mathematical Sciences (IMSc) in 2016. “High, thin clouds are often nearly transparent to incoming sunlight. They let it in rather than reflecting it, but then absorb the heat that radiates out from the Earth.” However, it is difficult to predict what the Indian monsoon cloud – the cumulonimbus – would do, she explains. “It is tall, starting at low altitude and reaching a few kilometers above, and can in principle both reflect and absorb.”
“Which is no shape at all, or every shape”
What is a cloud then? “When warm moist air rises from the ground, and it reaches the level at which water vapour will condense, that’s where you begin to see a cloud,” explains the late Roddam Narasimha, a fluid dynamicist and former Professor at the Department of Aerospace Engineering, IISc, in his talk given at the Science Gallery Bengaluru in 2020. The World Meteorological Organisation (WMO) defines it more literally, as “a visible aggregate of minute particles of water or ice, or both, in the free air.”
Back in the 17th century, Luke Howard named the three major forms of cloud as cumulus (heap), stratus (sheet) and cirrus (filament). And the fourth, a cloud containing rain, was named nimbus. Cirrus clouds are seen high in the sky. They are feathery and made up of icicles. Cumulus clouds start at a lower altitude but can grow tall and become bulbous. They are made of condensed water. Stratus clouds are sheets of either cirrus or cumulus clouds, and may be seen at different heights. Every cloud we see is one or a combination of these.
“To understand how clouds form, we need to understand the vertical temperature and humidity structure of the atmosphere,” says GS Bhat, Professor at the Centre for Atmospheric and Oceanic Sciences (CAOS), IISc. The atmosphere envelopes the planet, and has no upper limit – it ultimately leads up to space. The troposphere is the layer of atmosphere closest to the surface – about eight km at the poles to about 15-18 km at the equator – and this is where most clouds hang out. Very few move beyond, into the stratosphere.
Bhat, who studies rainclouds of the Indian monsoons, describes the shape of a tall cumulonimbus cloud. Such a cloud has roots near the Earth’s surface, from where water vapour rises as a plume – narrow at the root and wide above. As it rises and as the water vapour in it starts to condense, two things happen. One, the condensing part of the plume becomes visible as a cloud – with a nearly flat bottom demarking where condensation begins. Two, when vapour molecules condense, they release heat. If we assume that all vapour molecules condense, and account for all the heat that is released, then the difference in temperature at the base and at the top of a cumulonimbus could be 50ºC, earning them the name “hot towers”. “However, we must remember that the rising air expands and cools down, eventually consuming all the heat,” Bhat explains in his 2021 Resonance article “Clouds”.
Clouds in the lab
Clouds are shape-shifters. They are transient. Scientists have been sending sensors into them to detect what’s happening inside, but this can be dangerous because of turbulence and electrical activity. This has led them to attempt making clouds inside a lab.
One great leap in our understanding of clouds came about by pure chance; author Cynthia Barnett tells this story in her engaging book “Rain, a natural and cultural history”. Meteorologist Vincent Schaefer was working at the General Electric Research Laboratory in 1946. World War II was raging, and governments were pushing for the development of technologies to use in military tactics. For example, creating cloud-screens for hiding, or icing enemy aircrafts. But the scientist had had little success in triggering condensation of supercooled water vapour inside a cloud-chamber to form snowflakes or raindrops. Then one day, Schaefer threw some dry ice into a chamber in an attempt to cool it down further. Instantly, millions of tiny ice crystals appeared in the cold cloud inside the chamber. “We’ve got to get into the atmosphere and see if we can do things with natural clouds,” said his boss, Irving Langmuir, a Nobel laureate. They rented a plane, flew into a cold cloud over a mountain and dropped dry ice pellets into it. The cloud, writes Schaefer, “almost exploded” with ice crystals. This was how scientists figured out that minute solid particles, be it dry ice, dust or pollen, were crucial for inducing condensation of water molecules in vapour.
One great leap in our understanding of clouds came about by pure chance
Bhat also talks about a groundbreaking experiment carried out at IISc that addressed a baffling observation about a process called “entrainment”. A rising plume of cloud is turbulent, and has eddies of varying sizes. Entrainment is a process at the edges of any plume (smoke or cloud) where outside air is sucked in and mixed with the air inside the plume. The question essentially was how a plume of hot, moist air, rising from the Earth and climbing up to several kilometres does not just fizzle out. Smoke from a chimney or steam from a steamboat disappears within moments, but not clouds. Also, the height of a cloud did not fit the mathematical calculations. “If the entrainment was happening as we expected, then the height of a cloud should be half [of] what we observe, particularly for a tall cloud,” Bhat says. But nobody knew why.
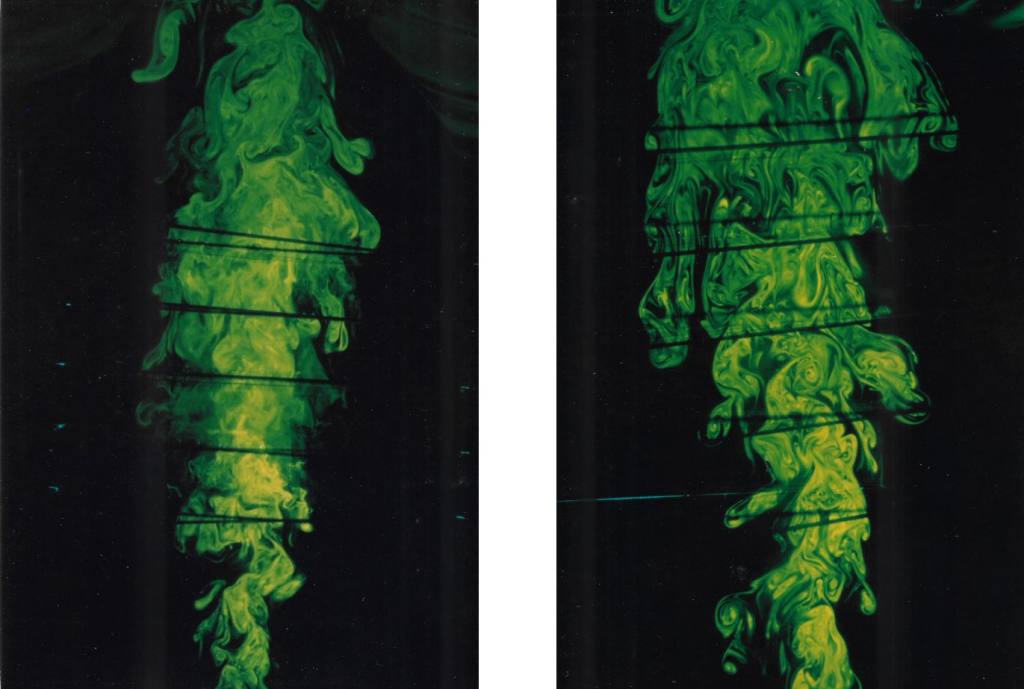
While entrainment was a well-studied process, Narasimha realised that those studies hadn’t accounted for the rise in temperature in a cloud as water molecules started condensing. Bhat and Narasimha decided to tackle this question by modelling a cloud in the laboratory. They modified existing technology to allow them to heat specific areas of the plume. The results were astonishing. Not only could they change the shape and density of a cloud by modulating temperature, they also found that entrainment near the base increased, resulting in the drawing in of air from the surrounding at this site, but there was hardly any entrainment in the middle and top parts of the cloud. It implied that in a natural cloud too, there is reduced entry of relatively dry mid-tropospheric air, which allows it to grow taller.
“How can I study from below, that which is above?”
There are urgent questions in cloud research, unanswered because of currently insurmountable hurdles. A world map created as part of a comprehensive study by the Royal Society predicting vulnerability to droughts and extreme flood events in 2090 has India blazing in colours with the highest numbers. “We are talking about advancing India in many other ways,” Rama surmises in her talk, “First of all, let’s make sure we have an inhabitable climate.” Numerous talks and articles stress upon how much we still don’t know about clouds. What makes cloud study so challenging?
An interesting if desperate appeal appears in an article published in Nature in 2015 – “Physicists, your planet needs you – climatologists highlight cloud mysteries in an attempt to lure physicists to their field.” It is undeniable that clouds are at the heart of our climate, and that they cannot be understood by applying any one area of science. Overcoming uncertainties in predicting climate needs every arrow in the quiver – from mathematicians and physicists to computer engineers, geologists and fluid mechanicians.
It is undeniable that clouds are at the heart of our climate, and that they cannot be understood by applying any one area of science
In one of his last talks given at the Science Gallery Bengaluru in January 2020, Narasimha explains what makes research on clouds so tough to tackle. Imagine a stream of fluid – anything that flows, be it liquid or gas. The smoke coming from a resting cigarette, or water running from a tap barely open. The flow starts out smooth, but then becomes turbulent. The smooth, laminar flow follows rules that scientists understand and can compute. The turbulent part of the flow however, is chaotic, irregular. “Even when the basic laws governing turbulent flows are known and have been known for 200 years,” Narasimha says, “turbulent flows remain largely ununderstood.” And clouds are turbulent flows.
Rama, in her talk at ICTS, also underscores a fundamental problem faced by cloud researchers. “Can we understand a simple, lone cloud?” She asks. “One small puffy object (a parcel of air) which is evaporating from the ocean, a little bit warmer and wetter than its surroundings; how does it climb a few kilometres and then condense to become a cloud? Even the most innocent looking cloud is often in violent turbulence. The water vapour, droplets, aerosols, all are moving within the large-scale eddies inside the cloud. Droplet thermodynamics contributes to this turbulence. Not all such puffs become clouds, but which ones do? How long will a cloud last? And which among so many will become a raincloud?”
“To predict anything about clouds, we have to solve incredibly complicated differential and partial differential equations,” she adds. “We know this, but we do not have the computing [power] required to resolve these. We cannot predict weather with great accuracy over long lead times using present day computers, and need much better instruments and much better satellite-based observations to get local weather right.”
Like all natural phenomena, our awe of clouds continues. Our dependence on them, the calamities they can cause, our ignorance about their nature, all add to the fascination we have for these floating objects, which appear so insubstantial, and yet are not.