How NMR is helping scientists probe molecular structures
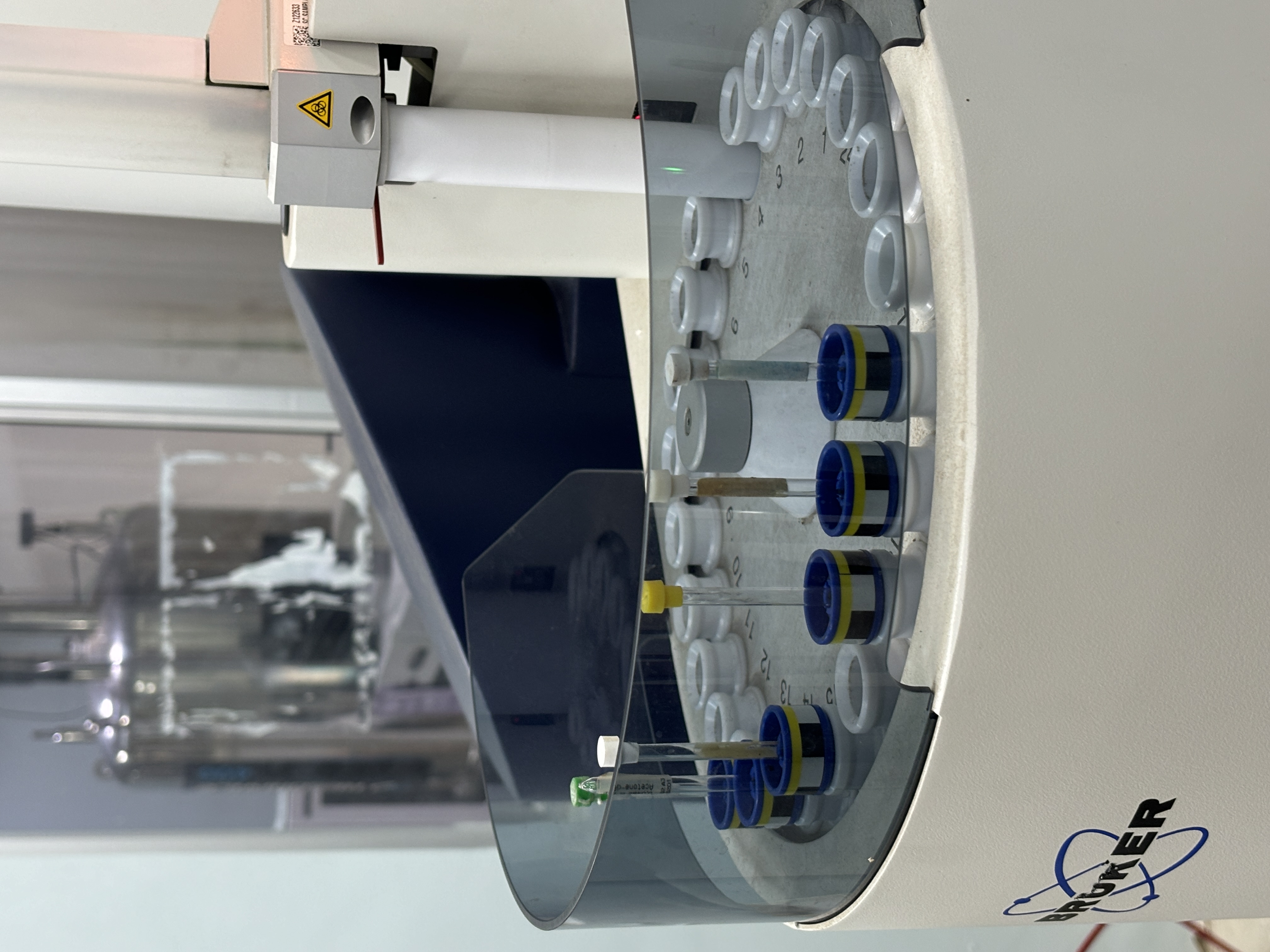
It was after six on a Monday evening in September, and Durga Prasad Hari was in a hurry. Holding a thin glass tube in his hand, he walked quickly to the common instrument facility on the ground floor of the Chemical Sciences building, two students tailing after him. After unlocking the door, he climbed up a small step stool and, with quivering hands, inserted the glass tube into a big, fat cylindrical machine. He pressed a few buttons, and the three of them waited with bated breath to see what would happen.
The machine they were using is a Nuclear Magnetic Resonance (NMR) spectrometer. It is an indispensable tool in unravelling molecular structures, be it organic compounds in synthetic chemistry or complex biological molecules like proteins. Anyone who has worked with NMR will tell you how frustrating it is to get a good spectrogram. But they would also be the first to tell you that the exhilaration of getting a flawless spectrogram makes the effort worth it.
Like other spectroscopic techniques, NMR tracks how light interacts with matter. The nuclei of many atoms, such as hydrogen, behave like tiny magnets. When placed in a strong magnetic field, these tiny magnets seek to align themselves along the field – like a compass needle pointing north.
When light falls on a nucleus, the latter gains energy, flips its orientation, and aligns in the opposite direction. However, the nucleus doesn’t like being in this “high energy” state, and quickly flips back to its original alignment, releasing the energy it gained.
The amount of energy released depends on the movement of its electrons and other atoms surrounding it. This means that each atomic nucleus within a molecule will respond to a unique frequency of light wave, called the resonance frequency. For example, hydrogen atoms in ethanol (CH3CH2OH) have a different resonance frequency compared to those in ethane (CH3CH3) simply because their molecular arrangement is different.
And that’s exactly what NMR tells us – it identifies the resonance frequencies of different atomic nuclei in a molecule, which appear as ‘peaks’ on a spectrogram. Every molecule will have a completely different NMR spectrum, which acts as a “fingerprint” of that molecule. This makes it a great method to conclusively determine the structures of organic compounds as well as biological molecules like proteins. The goal in synthetic chemistry is to get a “clean” NMR having all the peaks corresponding to the molecule, without any unwanted peaks which indicate the presence of impurities.
Every molecule will have a completely different NMR spectrum, which acts as a “fingerprint” of that molecule
“NMR is an instrument that we use in the lab daily,” says Hari, Assistant Professor at the Department of Organic Chemistry. It can help scientists estimate the efficiency of an organic reaction, map different spatial configurations of the same molecule, or study the kinetics of a chemical reaction. It can also help probe enzyme-substrate binding in biological systems, and design materials with specific binding capacities.
“For other techniques like X-ray crystallography, you need the compound to be a solid crystal,” explains Muhammad Arbaaz S, PhD student at the Materials Research Centre (MRC). “But NMR is so versatile and flexible that you can analyse compounds in the solid as well as solution phase. And it’s not just a preliminary test – it’s a very reliable confirmation of the identity and purity of a compound.”
Once, while analysing the NMR spectrum of a new class of molecules, Arbaaz stumbled upon something unusual. “The peaks in NMR are usually sharp, but I observed that this compound gave somewhat wavy peaks. I spoke to more people to understand what this might mean, and found that my molecule is magnetic!” Such materials with spontaneous magnetism have widespread applications in capacitors, memory devices, and even generating bioelectricity. Arbaaz excitedly adds: “I never expected that a strange observation with NMR would push me to explore an entirely different direction with my work.”
Probing fleeting proteins
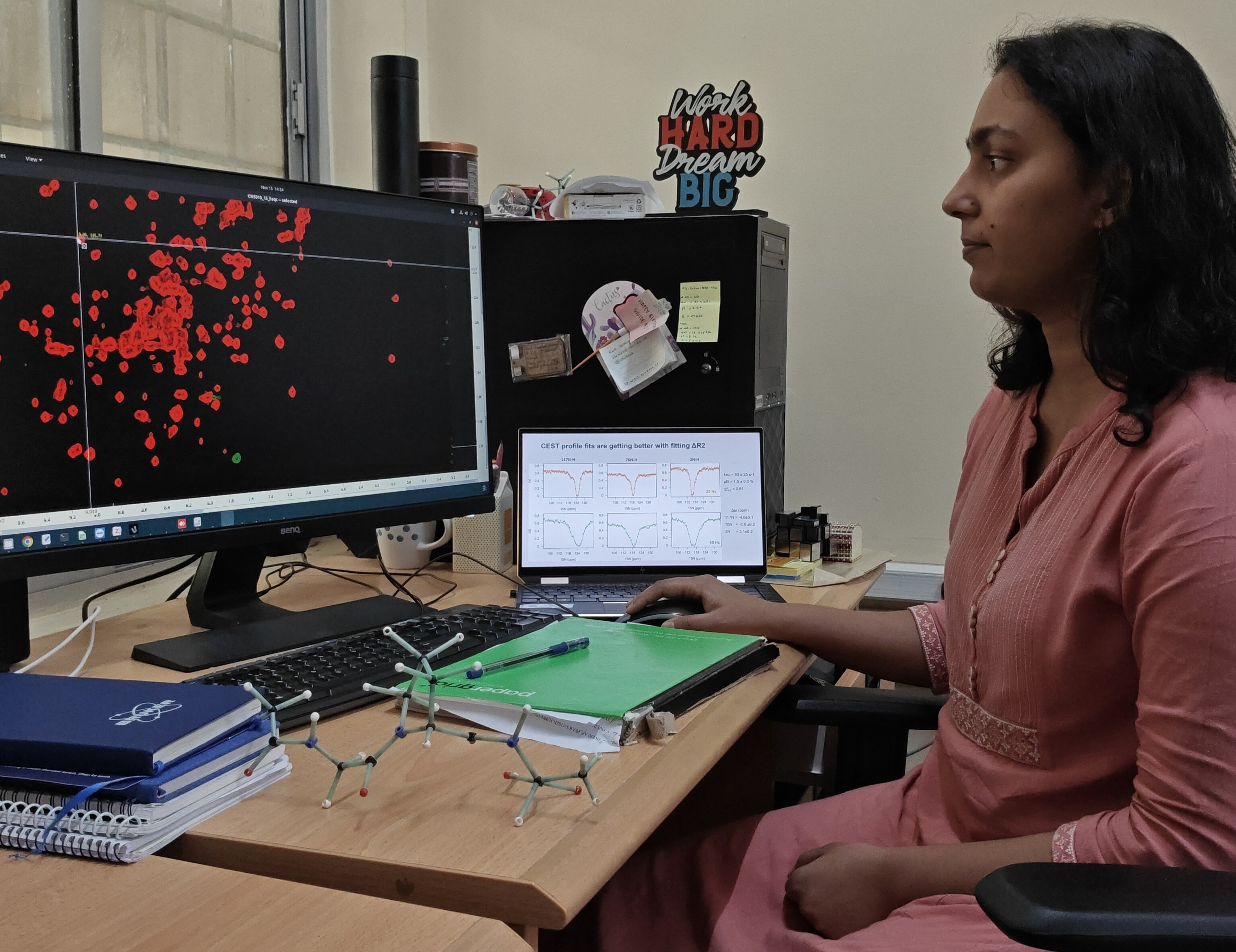
Across the road from MRC sits the Molecular Biophysics Unit (MBU), where structural biologists have been using NMR to probe biological phenomena, particularly how intrinsically disordered proteins (IDPs) work.
Unlike most proteins, IDPs do not have a stable, folded structure and adopt a variety of dynamic conformations. IDPs typically control biochemical processes by binding to partner molecules like DNA and other proteins. For most proteins, the structure dictates their function in a cell. So, how exactly do IDPs have specific functions without a fixed structure?
Ashok Sekhar, Associate Professor at MBU, investigated an IDP called cytidine repressor, which binds to DNA and prevents certain genes from performing their function. “We figured out that the disordered state of this protein is the one which [exists] around 90% of the time. But it transiently exists in a folded conformation with a millisecond lifetime – and we were able to characterise this state using NMR,” he explains.
Tracking this transient conformation is crucial because the cytidine repressor is only functional when it is folded. “It’s like trying to start your car with a piece of spaghetti, instead of a key with a well-defined shape,” adds Ashok. “Every once in a while, the spaghetti forms a car key, and at that time, the car is able to start. This was interesting because it was not known earlier that intrinsically disordered proteins can transiently fold even without the targets they bind to.”
Compounds studied by synthetic organic chemists usually have only a few dozen hydrogen atoms, but proteins are huge molecules, with several hundreds of these atoms. All the atoms are in a different chemical environment, surrounded by different neighbours, and each gives a distinct peak in the NMR spectrum. It becomes much harder to analyse the peaks, and it takes much longer to acquire the data. The best way is to leave the sample in the NMR machine and keep measuring the resonance frequencies again and again. This would allow it to catch new NMR signals that show up when there is a sudden and temporary change in the molecule’s conformation.
“Methods like X-ray crystallography have a harder time characterising fluctuations in structure – what we call molecular dynamics,” Ashok says. “But NMR is so powerful that it can detect states that have lifetimes of just a fraction of a second in solution.”
‘NMR is so powerful that it can detect states that have lifetimes of just a fraction of a second in solution’
NMR is already one of the most powerful techniques today, but people are working on making it even better and more extensive in scope – such as combining NMR with AI tools to hasten protein analysis.
“Using NMR to visualise larger biomolecules, accessing conformations with even shorter lifetimes, speeding up data acquisition and making it more sensitive – these are some of the things people are trying,” explains Ashok. “The other area that people are pushing is called in-cell NMR, where we can visualise molecules inside living cells using NMR spectroscopy. The possibilities are endless!”
An indispensable tool
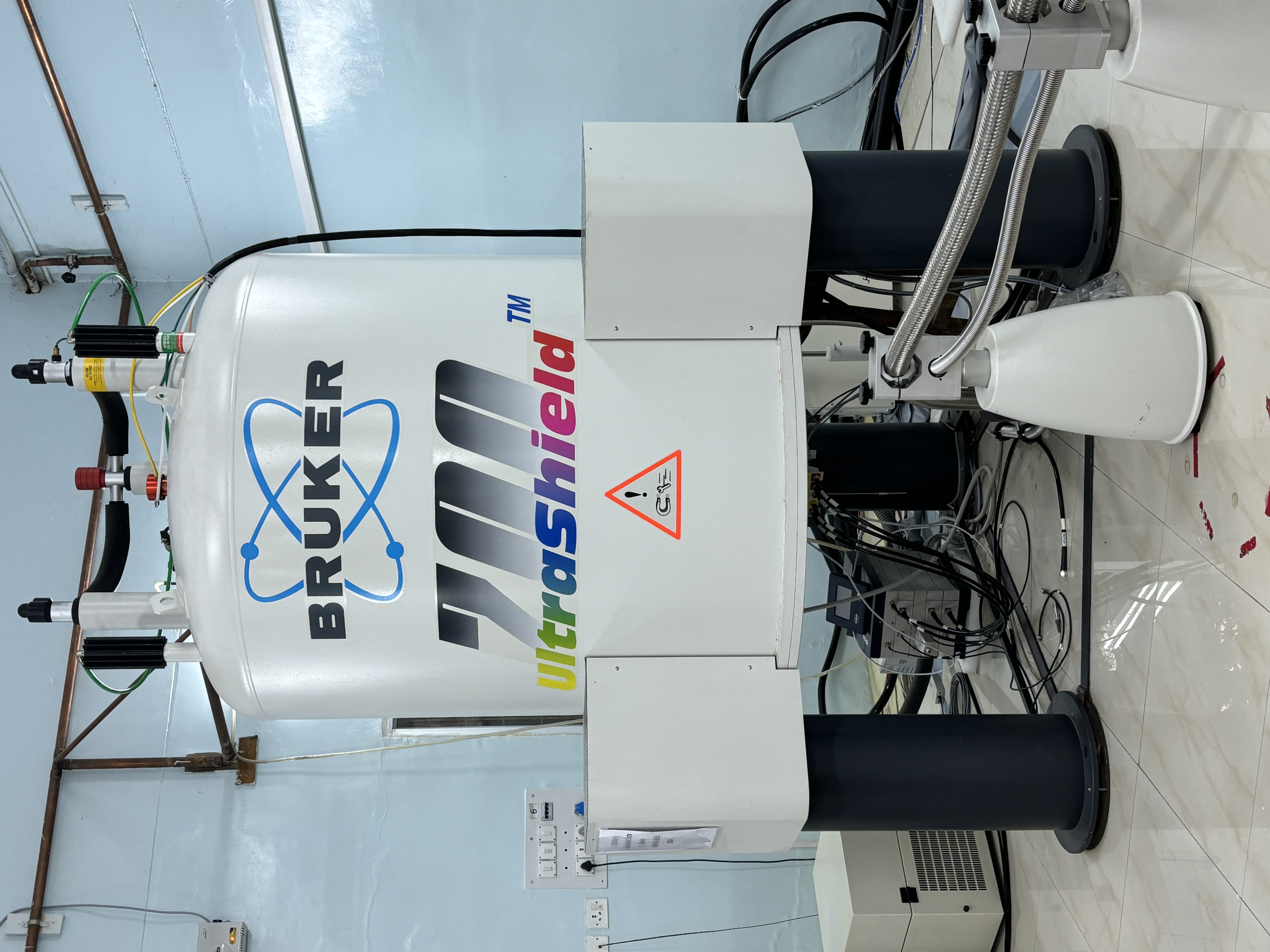
IISc has more than half a dozen NMR machines spread around the campus. The Chemical Sciences building houses four of them – two in the Department of Organic Chemistry, and two in the Department of Inorganic and Physical Chemistry. They look like something from a futuristic space settlement – sleek, cylindrical towers around three to four metres tall. Their huge magnets are kept cool using liquid helium and liquid nitrogen.
“There is, of course, a very strong magnetic field,” says Hari. “We are advised to not go near the NMR with any metallic objects when we go to load the sample. But we don’t stay there very long, so it’s safe.”
When the machine breaks down, a lot of work comes to a standstill. It is virtually impossible to move ahead in organic synthesis without getting a confirmatory NMR. This becomes a problem when your compound is unstable and may break down by the time you get the chance to run an NMR.
There are dedicated technicians in the department who run the samples for the students. But Hari explains that the machine is straightforward to operate, and it is possible for one to analyse their own sample. “Sometimes, when we have exciting results [or an unstable molecule] and the technicians aren’t there, I cannot wait for the next day. I always tell my students to give me the samples, and I run them on my own.”
Hari’s lab is working on a class of molecules called propellanes, which have three triangular rings of carbon atoms fused together, and look like a TV tower or a propeller. These are highly strained molecules – the angles between their bonds make them highly reactive. This reactivity can easily be harnessed to convert them into various other compounds. However, this also makes propellanes very unstable, and the NMR has to be checked immediately after separating the compound (which is done at -78°C). Even a slight delay may cause the molecule to degrade, Hari explains.
When patience pays off
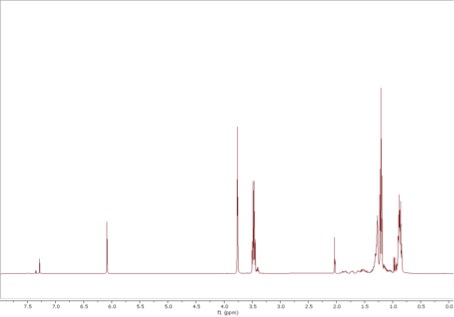
Two minutes felt like two decades to Hari, but the NMR sample he put into the machine was finally done scanning. As he and his students analysed the data, their excitement grew. The peaks in the NMR spectrum showed without doubt that they had successfully synthesised the propellane.
“When you get the NMR data and it shows you the peaks you were hoping for, it’s just like looking at your [exam] results in the10th grade!” Hari says.
Synthesising organic compounds is an incredibly long and tedious process, from setting up a chemical reaction to spending countless hours purifying and separating the compound of interest from an unknown mixture – which may not even have the compound! Researchers often have to carry out the same reaction again and again, varying different parameters like the amount of reactant, temperature and solvent, to figure out what works best. After weeks or even months of hard work, everything comes down to what the NMR analysis shows.
“The unsaid truth is that NMR invariably gives you a huge dopamine rush,” explains Arbaaz.
Once, Arbaaz had been trying out a particular reaction for a very long time, but failing to get the right spectrum repeatedly. “The incorrect NMR broke my heart every day,” he narrates. “Then I came across a new protocol for the reaction, and I got a beautiful NMR [spectrum] which matched perfectly. That’s the thing – NMR may disappoint you initially, but once you get it, it’s like you’re on top of the world.”
Arbaaz is now working on synthesising a new compound. Though he hasn’t got the expected results even after 20 times, he doesn’t plan on giving up. “Whatever happens,” he says, “I’ll keep working towards that NMR.”
Parth Kumar is a second year BSc (Research) student at IISc and a science writing intern at the Office of Communications