The small mouse has enabled giant leaps in biology research
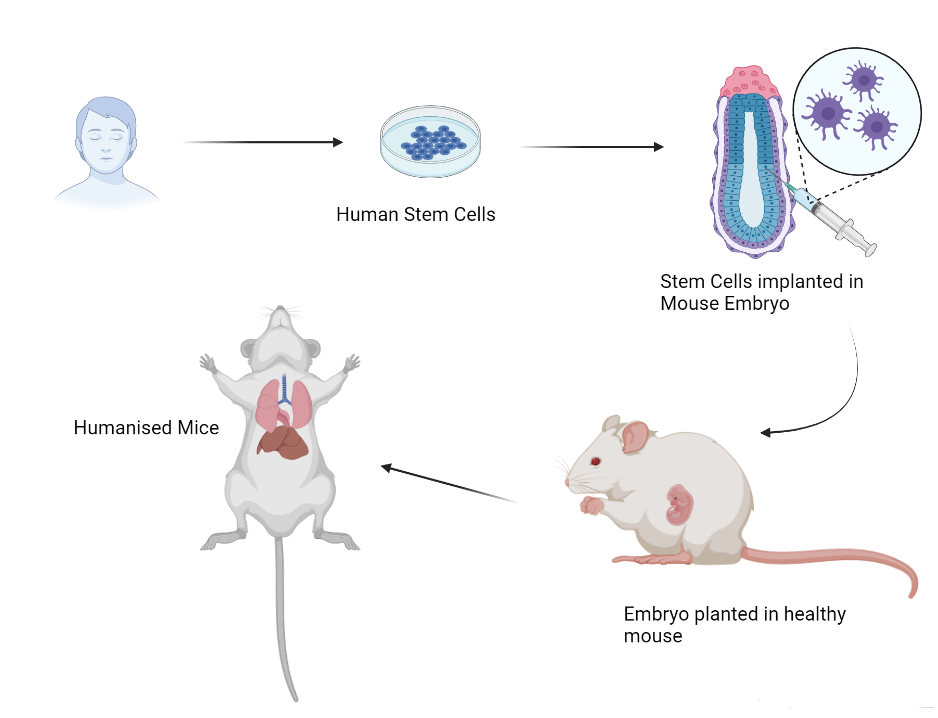
Claudius Galen (129-199 CE), a prominent physician in ancient Greece, obsessively studied anatomy – the science of how our bodies are structured and how our organs function. Since the ruling Roman government prohibited working with human cadavers, he dissected animals instead, to gain knowledge.
Even without human subjects, his investigations led to some remarkably accurate conclusions. One of the most important insights he gained was that the brain, and not the heart, controlled our actions. He deduced this when upon opening up the skull of a living cow and applying pressure to different parts of its brain, he could control its movements. He also found that muscles and nerves controlled our respiratory system, and kidneys produced urine.
Science has come a long way from the times of ancient Greece, and we have a deeper understanding of the human body. Still, there are many unanswered questions. Although now we can dissect corpses, researchers still need to work on live subjects to understand the normal functioning of the body. One of these subjects that has proved tremendously useful is the humble mouse.
A multifunctional model
Mice are magnificent model organisms. They are small, inexpensive to maintain, and easy to look after in a lab. Being mammals, they share considerable genetic similarities with humans.
According to the National Human Genome Research Institute in the US, on an average, the sequences of genes in the mouse and human genomes are 85% identical, with some genes being up to 99% identical. “Comparing mice to humans is complicated, but there are enough similarities to excite us to run experiments in these animals,” explains Rachit Agarwal, Assistant Professor at the Centre for BioSystems Science and Engineering (BSSE) at IISc, who is working on developing innovative drug-delivery methods. “By virtue of their genetic similarities, organ systems like the respiratory system, the immune system and others and their functioning are quite similar in humans and mice,” adds Ameya Dravid, a PhD student working with Rachit.
Mice are magnificent model organisms
With the advent of new technologies to manipulate genes, researchers unlocked a potential game-changer in the development and use of mouse models. Mice with new genes or DNA introduced into their genome are generally called transgenic mice. This technology can reduce the gap between mouse models and human systems.
According to the Foundation for Biomedical Research, more than 90 percent of all medical research labs in the world rely on the mouse model. “The mouse model has been in use for a long time; a large body of literature, genetic tools, methods, and reagents are available, making it a well-researched and easy to use model,” says Siddharth Jhunjhunwala, Assistant Professor at BSSE, who is interested in elucidating how our body reacts to foreign materials. A lot of knowledge can be gained from this model, especially now that we can engineer mice to express human genes.
But what is this knowledge, and how has it helped us?
Curbing contagion
Over the past couple of years, COVID-19 has wreaked havoc around the globe. Researchers everywhere have been working on understanding the disease and developing drugs and vaccines to counter the virus. One of them, Raghavan Varadarajan, Professor at the Molecular Biophysics Unit (MBU), is interested in developing vaccines against major viral diseases, primarily influenza, HIV, and COVID-19.
Influenza is a seasonal respiratory disease caused by viruses. Each year, the flu virus mutates, giving rise to new variants attacking the population. Current vaccine formulations target a viral surface protein with a highly mutable part. “That is why the vaccine needs to be updated annually,” remarks Raghavan. Using a mouse model of influenza, his lab has developed a vaccine formulation that targets a less changeable part of the viral surface protein. This might offer protection against a wider range of influenza viruses and reduce the number of flu shots that an individual needs to take in their lifetime.
The first step in creating a vaccine is to understand the interactions between the virus and our body. The COVID-19 virus, for example, infects human cells by binding to a cell-surface protein called the ‘ACE2 receptor’. Studying virus-ACE2 interactions in a test tube does not explain what actually happens inside our bodies. Using animal models like mice and guinea pigs has also been challenging since different animals have different versions of the ACE2 protein expressed in their cells. In addition, animal ACE2 proteins elicit different reactions to the virus compared to humans.
“Our colleagues at the National Centre for Biological Sciences (NCBS) have developed transgenic mice which express the human ACE2 receptor and can be used to study COVID-19 infections in mice,” says Raghavan. Transgenic mice that have human genes incorporated in them are called humanised mice, and they provide a great avenue to study interactions of virus and human proteins in the mammalian system.
Another issue with COVID-19 vaccines is refrigeration during transportation. It is likely that several batches of vaccines might be rendered unusable or unable to reach remote corners of the country due to a lack of refrigeration technology. Raghavan’s lab has developed a “warm” COVID-19 vaccine that is not just effective against all the major variant strains tested to date, but also does not require refrigeration for storage or transportation. This has huge implications for a country like India as well as numerous other developing countries.
Training memory
Mice are also valuable in neuroscience research.
Balaji Jayaprakash, faculty member at the Centre for Neuroscience (CNS), studies how memories are formed and how they help us to carry out daily tasks. “We don’t get to see inside the human brain at the microscopic scale, which makes it difficult to study the mechanistic causes of memory formation,” he says. His lab uses mice as a model to elucidate the
inner workings of the brain.
A recent study done in his lab suggests that our rodent cousins might be adept learners. In a related experiment, they investigate the effect of prior knowledge on problem-solving. The experiment involves presenting cues (flavour) to mice associated with specific locations in a sandbox. When presented with a certain flavour, the mice need to remember the associated hole and go to the correct location (one of the 5 white rings) to obtain the food reward.
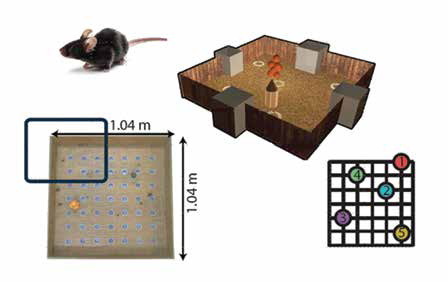
sandbox, location of cues and maze dimensions (Image courtesy: Balaji Jayaprakash)
They were able to show that the mouse can learn complex information – a set of five different flavour-place associations – but can learn faster when it is learning in relation to a prior experience or learning. If the second set is presented independently, many of them struggle to learn. On the other hand, when presented in relation to previously learned cues, they learn the new ones easily. Balaji says, “What would otherwise take them one and a half months to learn, they can learn in six days (if training is related to things they know).” More interestingly, they show that such learning is not only fast but also enables these mice to solve new problems. This may have implications in optimising the course structure in schools and colleges by emphasising and bringing out the relationships between concepts across the courses in adjacent semesters or adjusting the syllabus to build upon previous courses. On a smaller scale, this learning method can increase the amount of information one can learn in a short period of time.
Testing implants
When foreign particles enter our body, it assigns the immune system to fight against them. Although often life-saving, implants or prosthetic devices are also foreigners in the human body and elicit negative reactions from the immune system. Studies have shown that the first cells to attack an implant are neutrophils, which are the most abundant immune cells circulating in the body.
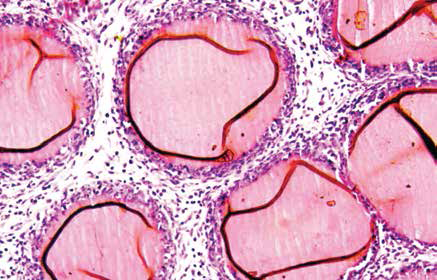
surrounded by immune cells (blue, purple).
(Image courtesy: Alakesh Singh)
The initial interactions between implants and neutrophils attract other cells and molecules to the site of implantation. These aggregate on the surface of the implant, leading to the formation of a capsule around the implant. This process is called fibrosis. Alakesh, a PhD student in Siddharth’s lab, studies this process in mice and is interested in quantifying the responses of the body towards different materials used to make implants.
Siddharth’s work on mice has helped identify key processes and players in the immune response. The hope is that this will help in engineering implants that are more compatible and better at evading our immune system.
Delivering drugs
Similarly, Rachit and his student Ameya use the mouse to study osteoarthritis, one of the most common chronic diseases that affect the joints. Ameya uses liposomes, nanoscale lipid capsules, as carriers to deliver drugs to specific joints in the body of the mouse. Liposomes carrying the appropriate drug are injected near the affected joints where they slowly deliver the drug.
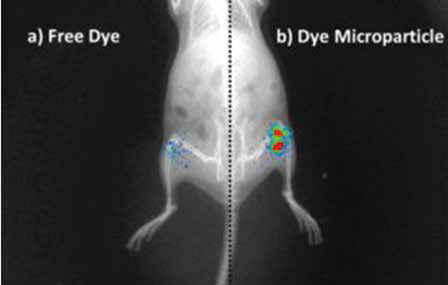
PLGA Microparticles (Image courtesy: Kaamini M Dhanabalan)
The lab uses another molecule, poly lactic-co-glycolic acid (PLGA), to create nanoparticles that can deliver drugs via an inhalant for respiratory diseases like tuberculosis (TB). These polymeric particles gradually reduce in size. “Once they become small enough they are either excreted by the kidneys or metabolised – used up to produce energy – by the body,” says Rachit.
Such drug-delivery methods in humans, if successful, might revolutionise modern medicine. Instead of two or three pills a day, just one of these injections once in a while may suffice.
Treading cautiously
Although Galen’s ancient experiments provided invaluable knowledge about the human body, they also produced or reinforced some misconceptions. He believed that blood is continuously produced by the liver and is used up by the body. His depictions of the human uterus were also more like a dog uterus. Galen probably discounted the differences in human and animal internal structures, and the lack of sophisticated technology might have played a significant role in amplifying his misunderstandings.
Today, researchers are aware of the risks as well as benefits of using animal models to study how the human body works. Scientists tread carefully by first establishing the similarities between humans and mice pertaining to their study, and then drawing conclusions based on insights from mice experiments. “The earliest experiments are to establish equivalence. Not equivalence in every aspect, but on a broad level,” explains Balaji.
Today, researchers are aware of the risks as well as benefits of using animal models to study how the human body works
Some fundamental dissimilarities are challenging to overcome. For example, mice are tetrapods (four-legged organisms) while we humans are bipeds (two-legged organisms). This means that there is a higher load on our joints compared to those of mice. Thus, diseases like osteoarthritis are more debilitating for humans and their progression is different.
Perhaps the most significant disadvantage of the model is something that also plagued Galen. Insights gained from mouse models, while very useful, are not necessarily directly transferable to human systems. “They are very useful models, but eventually these animals are different from humans, and hence additional studies are required for translation,” explains Siddharth.
Before any drug, device, or vaccine is certified for use, it often needs to go through an arduous process of testing in various animal models and human cells, starting often with the mouse. “Safety and toxicity studies in a couple of different animal models are needed to confirm that regardless of the protection a vaccine offers, the vaccine formulation is not toxic,” explains Raghavan.
Lately, there has been a push to completely eradicate the use of the mouse model and shift to working with human cells in Petri dishes or organs grown in labs. But this is often impossible in fields like neuroscience, where scientists need to relate brain activity to behaviour.
At the same time, there are ethical concerns and heavy restrictions on experimenting on higher organisms like monkeys or humans.
Given its far-reaching applications and well-established protocols combined with the number of researchers invested in using it, the mouse model is here to stay, at least for the foreseeable future. As Rachit puts it, “…The mouse model remains our preclinical gold standard.”
Arpit Omprakash is a PhD student at the Centre for Ecological Sciences and a former science writing intern at the Office of Communications, IISc