Self-organisation is the emergence of order in an initially disordered system and is observed from microscopic to astronomical levels
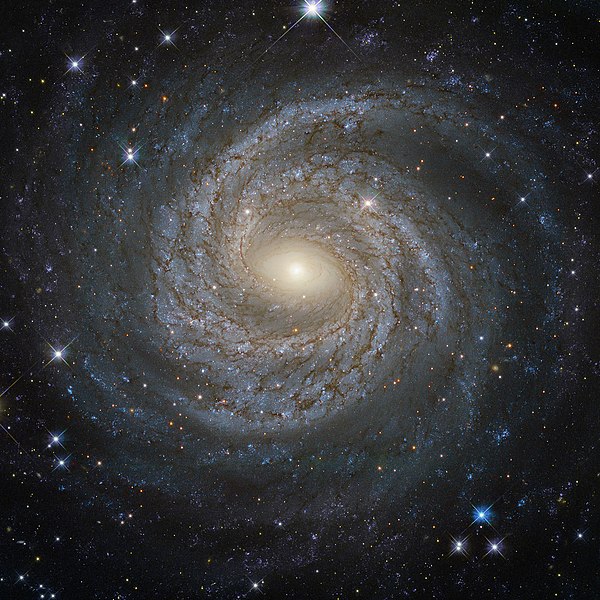
Spiral galaxies. Honey bees. Proteus mirabilis, the urinary-tract-infection-causing bacteria. What do they all have in common? A phenomenon called self-organisation. It transcends scale – from the formation of galaxies to the arrangement of lipids in cell membranes. Self-organisation is the phenomenon by which order is established in a system by the co-ordinated action of the smaller members of that system.
Self-organisation – an emergent phenomenon
Self-organisation exists in all fields. Nirupam Roy, Assistant Professor at the Department of Physics, who studies the formation of stars and galaxies, gives the example of the emergence of spiral patterns in galaxies. A galaxy is a collection of stars moving in their own orbits. All these orbits are oriented in a particular way determined by the large-scale gravitational forces among the stars. While the galaxies appear as spirals, the stars themselves aren’t arranged in a spiral pattern, as one would assume. Nirupam explains that every star takes a specific amount of time to travel in its orbit. If we consider separate times of revolution and orientations of orbits, then certain regions of the galaxy show higher density of stars at a time, giving it a spiral appearance. The formation of galaxies and their patterns follow a bottom-up or hierarchical approach, with smaller structures, like stars coming together to form larger patterns like spirals and ellipses.
The bottom-up approach is seen even in the regulation of societies of social insects like bees, wasps and ants. Raghavendra Gadagkar, the DST Year of Science Chair Professor at the Centre for Ecological Sciences (CES), has studied social insects for nearly 50 years. He differentiates between the two types of control seen in these insect colonies – bottom-up and top-down. In top-down control, there is one leader who knows how a particular task must be carried out and not only communicates it to the others, but also makes sure that everybody fulfils their role. On the other hand, bottom-up control involves no leader. The tasks of the colony are regulated in a decentralised manner. In his article More Fun Than Fun: The Exquisite Wax Palace of the Honey Bees, Raghavendra throws light on how a large number of bees perform simple tasks following basic local rules, ultimately leading to completion of complex tasks like building the hive. This is an example of self-organisation.
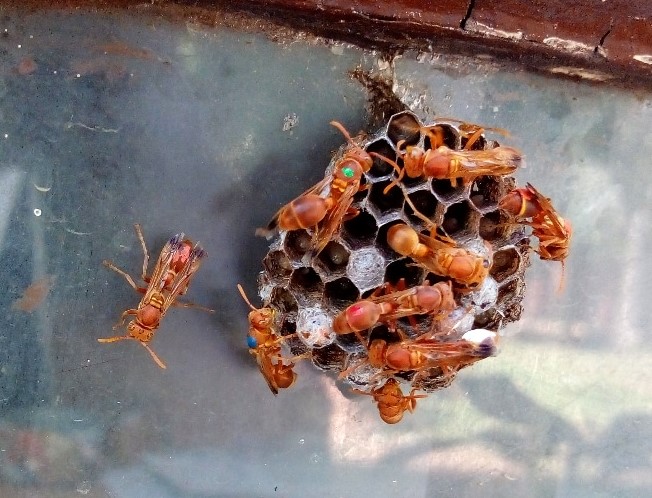
and Policy, IISc (Photo: Anoushka Dasgupta)
Self-organisation leading to collective animal behaviour depends on the balance between the costs and benefits to the members of the system. Vishwesha Guttal, Associate Professor at CES, highlights the case of predators. “While a predator can easily spot groups of prey, and may manage to hunt some individuals, the chance of each individual getting caught reduces as the group grows larger.” Community living is advantageous for unicellular organisms too. Varsha Singh, Associate Professor at the Molecular Reproduction, Development and Genetics department works on swarming bacteria. She explains, “If bacteria want to punch holes in a eukaryotic cell, a single bacterium may keep trying to punch holes, yet not succeed. But when they unite, they are more likely to succeed and receive nutrition [and propagate].”
Driving forces behind self-organisation
We know some of the driving forces behind self-organisation in several systems. In social insects, the complexity of the tasks and the cognitive ability of individuals determine whether the top-down or bottom-up approach is used. For example, termites make large, complex mounds. “It’s very hard to imagine that a queen termite has a detailed architectural map in her brain, or be able to communicate to the workers exactly what to do. They have tiny brains – the size of a pinhead – but they accomplish very complex tasks,” elucidates Raghavendra, concluding that self-organisation is at play in such cases.
The complexity of the functions to be carried out by certain structures like the plasma membrane also dictates self-organisation. The plasma membrane has more than 800 species of lipids. Lipid molecules are asymmetric, with a distinct water-loving head and a water-repelling tail. Prerna Sharma, Associate Professor at the Department of Physics, who works on membrane biophysics, explains that the tails of lipids are always oriented away from water, and their heads always face water, making the system energetically stable.
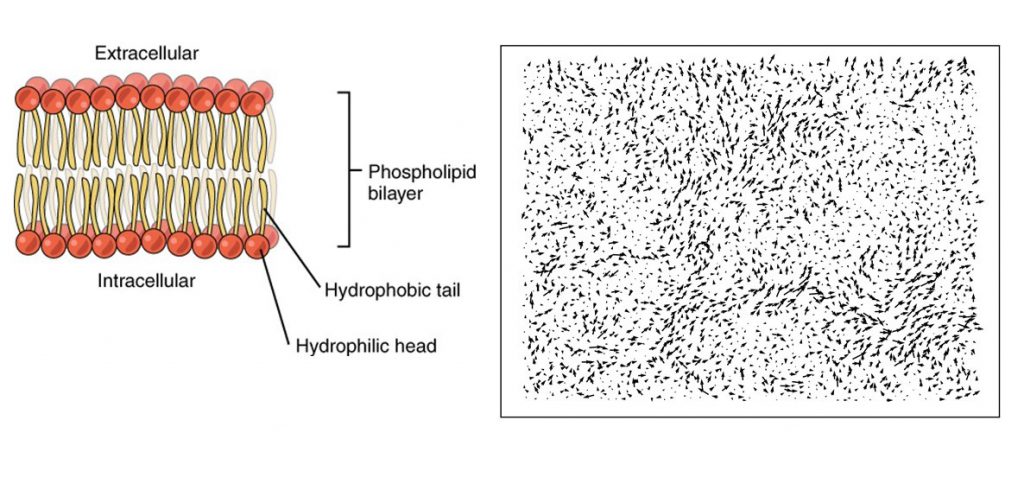
Right: Tracking movements of lipids in a membrane over time using
molecular simulations (Image courtesy: Harini Sureshkumar,
Molecular Biophysics Unit, IISc)
While this orientation of lipids is uniform, the composition of the species of lipids in different regions of the membrane may vary. “Since there are so many different kinds of lipids, there is also a huge number and types of interactions among them, leading to a complex organisation,” describes Anand Srivastava, Assistant Professor at the Molecular Biophysics Unit. This raises questions like what determines the composition and organisation of lipids in the plasma membrane, and how their organisation affects their functions.
Other than the forces that drive self-organisation in complex systems, there are also forces that hold several systems together. At the gargantuan scale of space, stars emerge from dense molecular clouds. “At an intermediate scale, there is an interplay of thermodynamic processes like heating and cooling, which depend on the density and temperature inside the cloud. These in turn are affected by magnetic forces and large-scale gravitational forces,” explains Nirupam.
Studying the physics behind self-organisation
Lipids are the building blocks of the cellular plasma membranes, but they are hard to visualise and work with. So instead, Prerna’s lab uses rod shaped M13 viruses mixed with polymers like polyethylene glycol. In such a mixture, as reported by The Life of Science, the viruses that Prerna studies organise themselves into a single layer, with physical properties similar to that of plasma membrane. “There is an attraction between the rods in a way that the alignment of the rods is preferred over random orientation,” says Prerna. This system is larger, and easier to visualise and tweak to study membrane biophysics. Her breakthrough work is in the area of stability of the plasma membrane.
Another researcher using asymmetric particles to study self-organisation is Ajay Sood, Year of Science Professor at the Department of Physics, in collaboration with Sriram Ramaswamy, Professor in the same department. Unlike Prerna, they use inanimate particles – brass rods, around four mm long with a physically distinct head and a tail region. These particles are placed on a vertically vibrating plate. The vibrations from the plate act as the ‘fuel’ for the particles to move. Systems whose individual particles use energy and move are called ‘active matter’. The direction of the movement of the brass rods is determined by their orientation. Over the past 10-15 years, Ajay’s lab has made several important discoveries about the collective behaviour in this non-living system. They found that a minimum number or quorum was required for the rods to show cooperative movements. Also, a coordinated movement was observed when inactive spherical beads were interspersed between them, which act as a medium of communication. Expanding on the concept of active matter, Ajay adds, “Systems like insects, birds, fish, etc, are all examples of active matter.”
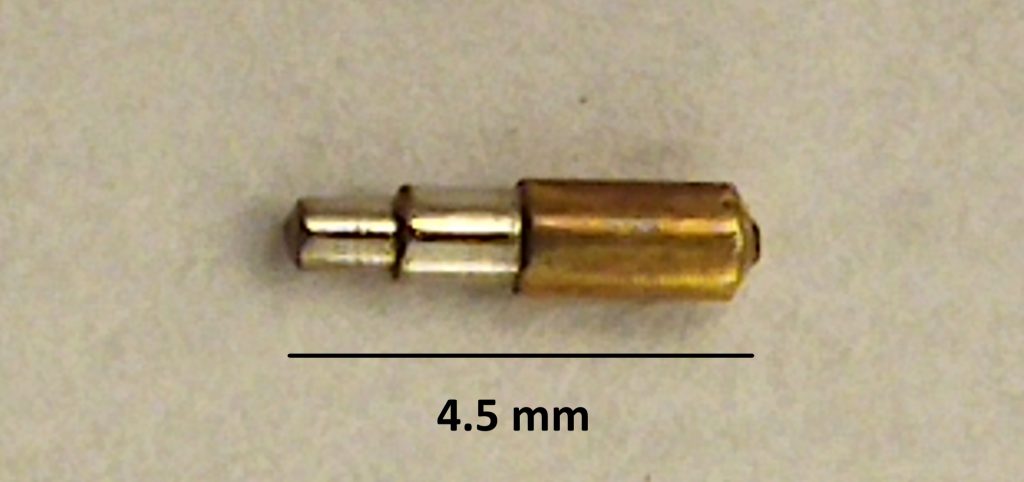
under certain conditions (Image courtesy: Raushan Kant,
Department of Physics, IISc)
Active matter is governed by nonequilibrium physics. The vastness of this concept is beautifully explained by Sriram. “Anything you see around you that’s alive is in a nonequilibrium state,” he explains, adding, “By nonequilibrium, I mean a thermodynamic parameter, like temperature, or chemical potential is maintained at different values for different components or locations. This will always result in a flux of energy through the system. And all life [is maintained by] converting this flux of energy into work.”
Sriram, a condensed matter physicist, studies the organisation of different states of matter and the rules governing them. His journey into biological physics and the formation of flocks in organisms stems from observing common properties between living systems like bacterial or locust swarms, and liquid-crystalline states. One of his research interests is finding the rules at the heart of the behaviour of motile organisms moving through a viscous fluid medium.
The plasma membrane is also in a state of nonequilibrium. Anand explains, “While a complex system tends to go towards an equilibrium level, the constant disturbances in the plasma membrane do not let it stabilise. Therefore, there is always a state of flux.”
‘Phone a friend’ in case of disturbances
Self-organisation depends on a feedback system as observed in fish schools and blackbuck herds by Vishwesha’s group. He gives the example of predator attacks as an external stimulus in blackbuck herds. At first, a few members of the herd may spot a predator and start moving to avoid it. Due to an intrinsic feedback mechanism, their neighbours start to copy their movement. In this way, the reaction to move away from the predator is amplified through the herd and they are able to escape the predators with minimum damage. Thus, the interplay between the external stimulus and internal feedback helps the herd escape.
Communication and feedback are key to every activity of cooperative insects like honey bees and wasps too. Colonies of the paper wasp, Ropalidia marginata, show a division of labour based on factors like age and dominance. Different members perform specific tasks like foraging, looking after the larvae and building their nest. Raghavendra says, “My students have shown that in R. marginata, if the workers in the nest receive food from the forager without showing dominance behaviour [like biting], then she knows she doesn’t need to bring any more food. Whereas if they bite her and snatch the food, it means that more food is required.” This communication is a feedback for the foragers. Honeybees use a less aggressive feedback system. “Foragers bring food and nectar and have to wait in a queue for the food to be unloaded. If they wait very long, they understand that not much food is required. But if they are mobbed and the food grabbed from them, they know more food is required. So, the longer the queue, the less the food requirement,” says Raghavendra.
Not only animal systems, but plants growing in clumps in semi-arid areas or grasslands also show cooperative behaviour and a feedback system. Self-organisation in in plants is in the form of collective growth. Vishwesha says, “The presence of one plant facilitates the other plants nearby to grow better.”
Not only animal systems, but plants growing in clumps in semi-arid areas or grasslands also show cooperative behaviour and a feedback system
Perturbations in these self-organised systems are usually short-lived. Be it in the ephemeral disturbances seen in stars and galaxies, or be it predator attacks on blackbuck herds; complex systems go back to their original order. Sometimes there are obstacles in the path of collectively moving groups. Swarming bacterial colonies spread in different patterns on a moist and nutrient-rich surface. They swarm around obstacles, as seen by Varsha’s work on Pseudomonas aeruginosa. “We believe that the bacteria produce a chemical signal, which helps them realise that there’s an obstacle close to their moving end, and they should change direction,” says Varsha. Her team thinks that the signal might be a chemical molecule that diffuses from bacteria through the surface, and is recognised by sensors on the other bacteria.
Sriram’s team of physicists has also modelled the movement of ‘swimmers’ in a viscous medium. One of his key findings is that it is impossible for large numbers of slowly swimming creatures, like bacteria, to align in the same direction. They dissolve into a chaotic state called ‘bacterial turbulence’. Purely physics-based modelling of collective behaviour, however, gets increasingly difficult with increasing complexity of the organisms and the motivation behind their actions.
Some kinds of obstacles can cause more long-lasting disturbances in flocks. Ajay’s group studies what would happen if you mix active matter, in the form of self-driven brass rods that are prone to flocking, with other moving particles that are averse to flocking. He equates it to putting moving pillars in a crowded space with humans, which compels them to break the ‘flock’. These anti-flocking particles, called ‘dissenters’, disturb the flocking of the other particles as well. “It’s a case where birds of different feathers don’t flock together,” he quips.
Self-organisation of living and non-living matter and their constant state of flux is evident everywhere. Deciphering the rules governing it is vital to understanding how the very fabric of the universe works in a constantly changing state. The study of active materials is as dynamic as these systems themselves. The essence of this is captured by the American biophysicist Rob Phillips when he explained the clever title of his review from 2015, Napoleon is in equilibrium, which implied that only dead things are in complete equilibrium.
Anoushka Dasgupta is a freelance science writer and former intern at the Office of Communications, IISc. She has a Master’s in Biotechnology from Savitribai Phule Pune University