Seismologists have their work cut out when it comes to understanding and predicting earthquakes and their impact
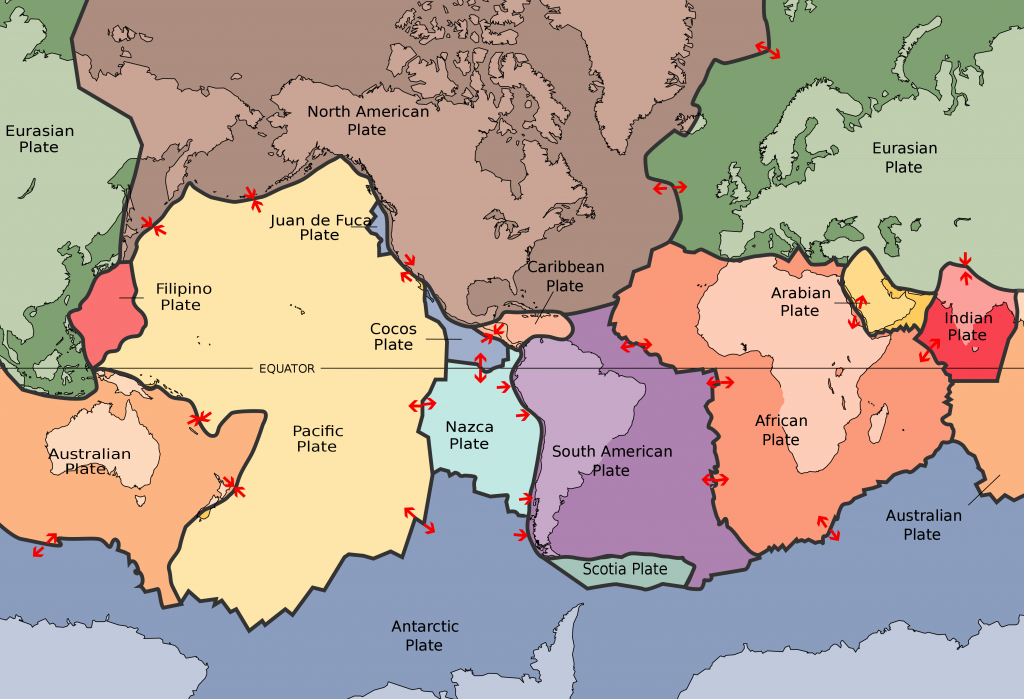
(Image courtesy: Scott Nash/Wikimedia Commons)
Beneath the serene snow-capped mountains of the Himalayas, danger lurks. Scientists are increasingly worried about a big earthquake overdue in the Himalayan region, anticipated to have a magnitude greater than 8.0 on the Richter scale. To give some context, the 2015 earthquake in Nepal had a magnitude of 7.8, resulting in 9,000 deaths, 22,000 injured, and the flattening of large parts of Kathmandu city. One can only imagine the scale of damage that the predicted Himalayan quake would inflict.
“Himalayas is an active plate boundary where the two plates (Indian plate and Eurasian plate) are converging at about 20 millimetres per year,” says Kusala Rajendran, former Professor at the Centre for Earth Sciences (CEaS), IISc. She explains that the rocks in these areas are weak and under stress due to this collision of plates, which is why earthquakes are common in this region. But what are these plates?
The Earth’s lithosphere (the crust and the upper mantle) is brittle and broken into massive, irregularly shaped interlocking slabs known as tectonic plates. Attreyee Ghosh, Associate Professor at CEaS, explains that the Earth can be imagined as a chocolate with a hard outer crust and an interior filled with gooey matter. Now, if a fine knife cuts the crust without taking the pieces apart (still intact as a sphere), the resulting pieces of the hard shell are representative of tectonic plates.
Although we can’t see it in action, the land below us is in constant motion and has rifts and drifts. When two plates come close to each other, the interactions between their boundaries can create high mountains or deep trenches and, more often than not, earthquakes. The Himalayan plate boundary is active because of the way it was formed. “The Indian plate was in the southern hemisphere, close to Antarctica. Suddenly, it just broke away and accelerated northwards to the current position north of the equator,” says Attreyee. This rapid northward motion and its collision with the Eurasian plate, around 40-50 million years ago, formed the Himalayas. While this might be common knowledge, the reasons for the plate’s fast movement are not well understood. Attreyee believes that the acceleration of the Indian plate could be attributed to the Reunion plume – an active volcanic hotspot in the southern Indian Ocean, which is thought to be responsible for the eruption of the Deccan Traps, one of the largest volcanic structures on Earth. Plumes are massive eruptions of hot, low-density material from the mantle, and the Reunion plume is believed to have been active for over 65 million years, last erupting in December 2020.
Attreyee and her team employ physics as a tool for time-dependent mathematical modelling – they consider the Earth’s configuration millions of years ago and simulate specific conditions leading to its current state. They modelled the Indian plate close to Antarctica, in the state that it was millions of years ago, and simulated its interaction with the Reunion plume. “The plume was able to remove the base of the Indian plate, possibly contributing to the anomalously low thickness [of the plate]; the reduced thickness and thereby the reduced mass of the plate potentially aided in the Indian plate’s sudden acceleration,” says Attreyee.
Earthquakes are also observed in stable regions away from plate boundaries, like in Latur in 1993 and Bhuj in 2001. Kusala explains that tectonic activity millions of years ago has left some residual stresses even in such stable landmasses, and old faults get reactivated, leading to earthquakes. “When seismic waves from a plate boundary translate through the plate interiors, the waves simply dissipate, but in regions with a fault, these waves can amplify and trigger earthquakes. Somewhat like rubbing on old and healed wounds, yet subject to pain if activated,” she says.
“Earthquakes originate several kilometres (ten to hundreds) below the surface, and it is hard to make direct measurements of the factors that cause them”
While earthquakes in active zones like the Himalayas are common, those in stable regions are very infrequent. Estimates are uncertain, but they typically occur once in hundreds or thousands of years. There has been no significant earthquake in Latur prior to 1993 and none after that (as far as experts know). Kusala remarks that the extended time gap between these events in such regions leads to a false sense of security among people. With no prior experience of earthquakes, buildings are not designed to withstand them, leading to disproportionate damage.
A question that is frequently posed to Kusala is about the unpredictability of earthquakes. “In predicting the weather, for example, all the constituent elements – humidity, temperature, cloud cover, wind speed – are measurable, yet the weather can only be fairly predicted. But earthquakes originate several kilometres (ten to hundreds) below the surface, and it is hard to make direct measurements of the factors that cause them,” says Kusala. So, what is the best way to deal with the situation? With the information currently on hand, scientists are able to map the faults, identify their potential, predict the maximum magnitude expected, the extent of ground shaking and even suggest a time window. “However, we still cannot fix a date,” explains Kusala.
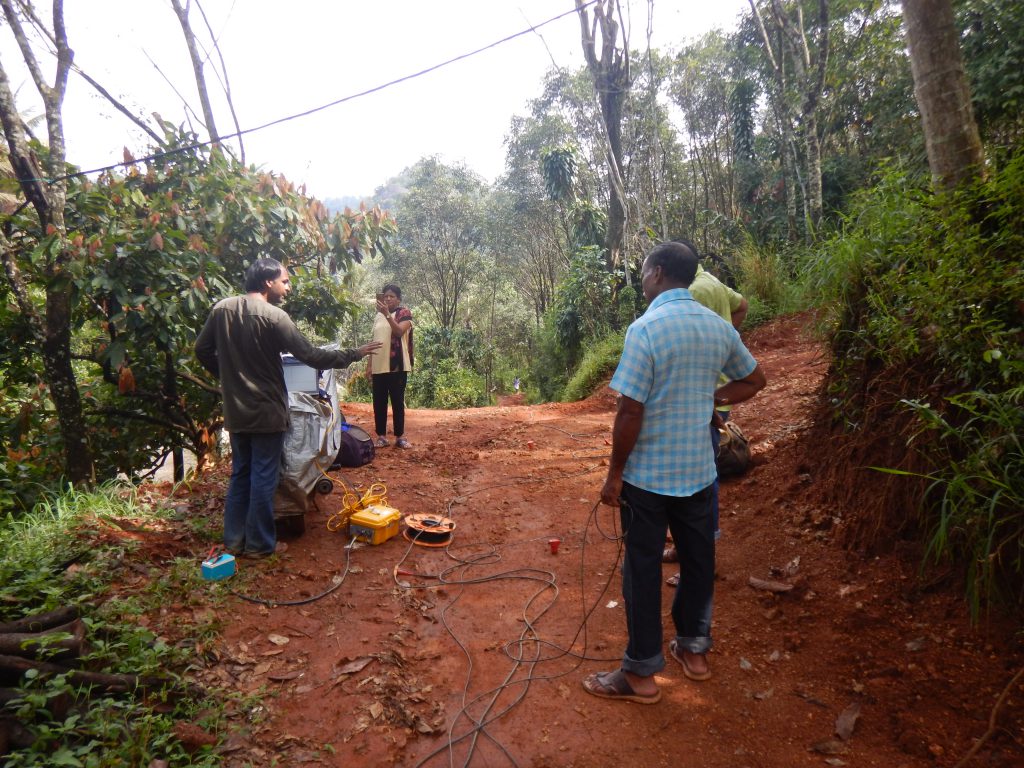
Better safe than sorry
Given the unpredictability of earthquakes, the only option is to be prepared for them. Structures like nuclear power plants can be constructed far from known earthquake faults. But bridges, dams and hospitals are needed everywhere. Understanding the nature of earthquakes and designing structures to withstand them is essential, says Swetha Veeraraghavan, Assistant Professor in the Department of Civil Engineering at IISc.
Swetha, along with her collaborators, had previously developed an open-source simulation tool to determine the response of a structure to an earthquake of a specific intensity. The output of the simulation – potential stresses and strains in the building – can be used as parameters in designing the columns and beams. Swetha’s team at IISc is now augmenting the tool with additional features. She adds that other than making changes in the design, the influence of earthquakes can also be reduced by using isolators – dampeners made of rubber and lead – at the base of the structures to dissipate the waves.
“We work on the seismic safety of structures like underground tunnels and dams, and on metamaterials development,” says Swetha. She explains that a metamaterial is a composite fabrication in which different materials are placed in a periodic fashion to give it the ability to weaken the impact of an earthquake. When placed around a structure, these metamaterials shield it from the seismic waves.
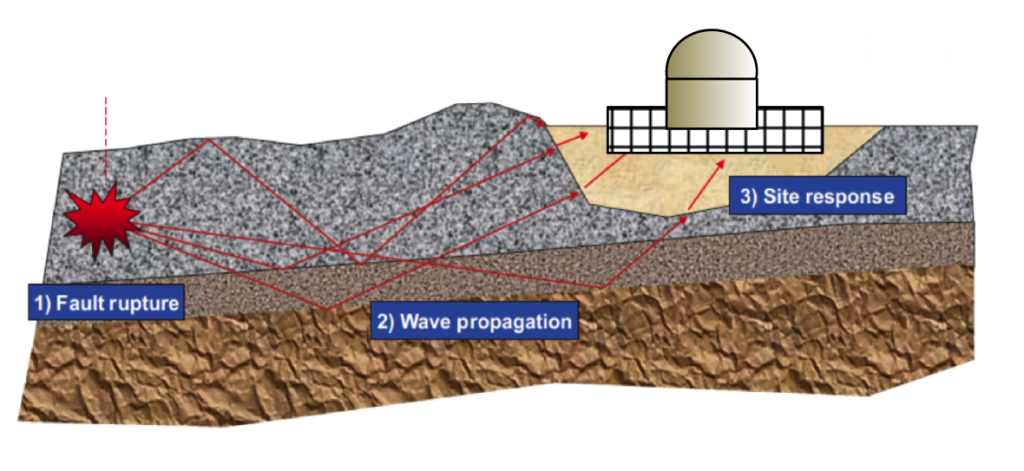
The design of an earthquake-resistant dam is challenging as there is a combination of water and soil – “a two-phase system,” remarks Swetha. The water causes the dampening of earthquake waves in some cases, but beyond a certain threshold, the earthquake can potentially reduce the strength of the construction material leading to massive destruction.
Currently, only a few parameters like soil properties at varying depths are taken into account for designing civil structures. But for efficient seismic design, other factors also have to be taken into account. India is divided into four seismic zones and each zone is assigned a specific earthquake intensity. “And all structures are designed based on the zone-specific earthquake intensity without considering the proximity of the earthquake fault to the structure and the complexity of the wavefield incident on the structure,” remarks Arun M George, a PhD student in Swetha’s group. Unlike these traditional approaches, he designs dams after considering their shape, the soil type (which varies with the location), and a range of such site- and structure-specific parameters that would make these structures more efficient in withstanding earthquakes.
Seismology – the study of earthquakes and the movement of related waves through the Earth – is not always about death and destruction. Swapnil Macche, a project associate who works with Kusala and her collaborators, applies machine learning techniques to analyse data from geophysical explorations to locate oil or gas fields. These explorations involve setting up artificial micro-explosions to induce controlled earthquakes at a source point. These explosions trigger pressure waves, and the readings of these waves are taken at different locations in the site of interest. A variation in pressure waves indicates a change in the composition of the bedrock and also points to the presence of oil or gas fields. “In the available approaches, only 30% of the explorations result in identifying an oil or gas field; that’s a lot of money wasted,” says Swapnil. Swapnil’s research focuses on using algorithms to obtain sharper signals to identify the oil and gas fields.
Is our Earth in shape?
While Swapnil explores the domain of oil and gas fields within the earth, Debanjan Pal, a PhD student in Attreyee’s research group, focuses on the Earth’s overall shape.“If we see the shape of any planet, it is ellipsoidal (egg-shaped), but through satellite images, geologists have observed the Earth to be potato-shaped,” he says. Irregularities in the Earth’s shape are referred to as geoid anomalies.
If the Earth were made of just one material, gravity would pull the surface uniformly, resulting in a perfect ellipsoid. But, when our planet started to cool after its formation, the heavier material sank to the core and the lighter material moved up towards the surface. In addition, other processes within the earth, like thermal convection (transfer of heat due to movement of liquid matter), stirred up the constituents resulting in a very non-uniform distribution of mass. Since the effect of gravity is directly related to the mass of an object, this uneven distribution leads to the irregular shape of the Earth, explains Debanjan.
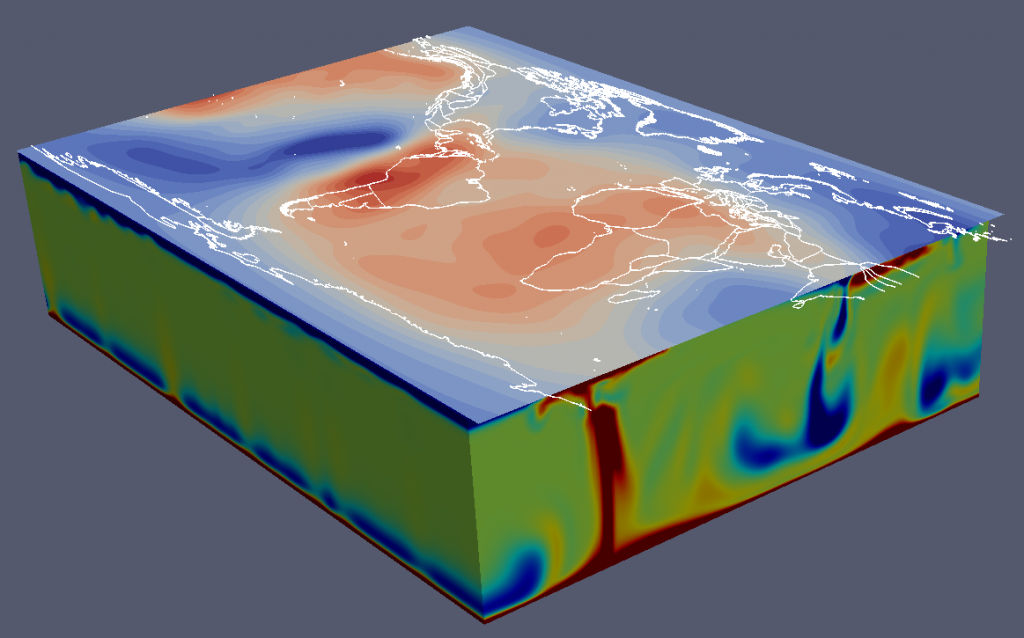
Debanjan’s work focuses on the genesis of the Indian Ocean geoid low, a 106-metre depression in the seabed and the lowest gravity or geoid anomaly on Earth. He employs numerical techniques to compute the Earth’s mass distribution over time – starting from 140 million years ago. One of his preliminary findings is that the African superplume caused a mass deficit within the mantle, indirectly bringing about the observed low gravitational anomaly in the Indian Ocean.
Breaking down the science of quakes
One of the critical challenges researchers face in studying the Earth and earthquakes is limited data availability. Physical measurements hundreds of kilometres below the surface are unfeasible and obtaining data to train Swapnil’s machine learning tool or validate Debanjan’s theory is difficult.
In addition, the study of earthquakes, Kusala believes, is complete only with observations in the field. “Speaking to the local people, exploring the area, and digging up the history provides valuable insights into earthquakes beyond the data recorded by instruments,” she says. She recollects post-earthquake surveys in Chamoli, Uttarakhand, in 1999, after an earthquake of magnitude 6.3 hit. At the Gopinath temple at Gopeswar, where the earthquake had created minor cracks, the priest pointed to damage from a massive 1803 event in the Garhwal Himalayas. And the evidence? Recycled bricks with misaligned and inverted inscriptions in Devanagari. Here was proof that a larger earthquake can level the structures. “Historical data helps to frame the window, or the time elapsed since the last earthquake,” she explains.
“Speaking to the local people, exploring the area, and digging up the history provides valuable insights on earthquakes beyond the data recorded by instruments”
Due to her vast experience in seismology, students, young researchers and science journalists often flock to Kusala to get clarifications about various aspects of earthquakes. She is now co-authoring a popular science book on earthquakes with CP Rajendran, an adjunct professor at the National Institute of Advanced Studies. The book’s narrative is packed with anecdotes from her years of fieldwork, she says. “Our [Kusala and C P Rajendran] USP has always been trying to relate what you see today to what could have happened in the past.”
Mohammed Asheruddin is a PhD student at the Centre for Sustainable Technologies, and a science writing intern at the Office of Communications