The study of model organisms is the key to unlocking mysteries about life on earth
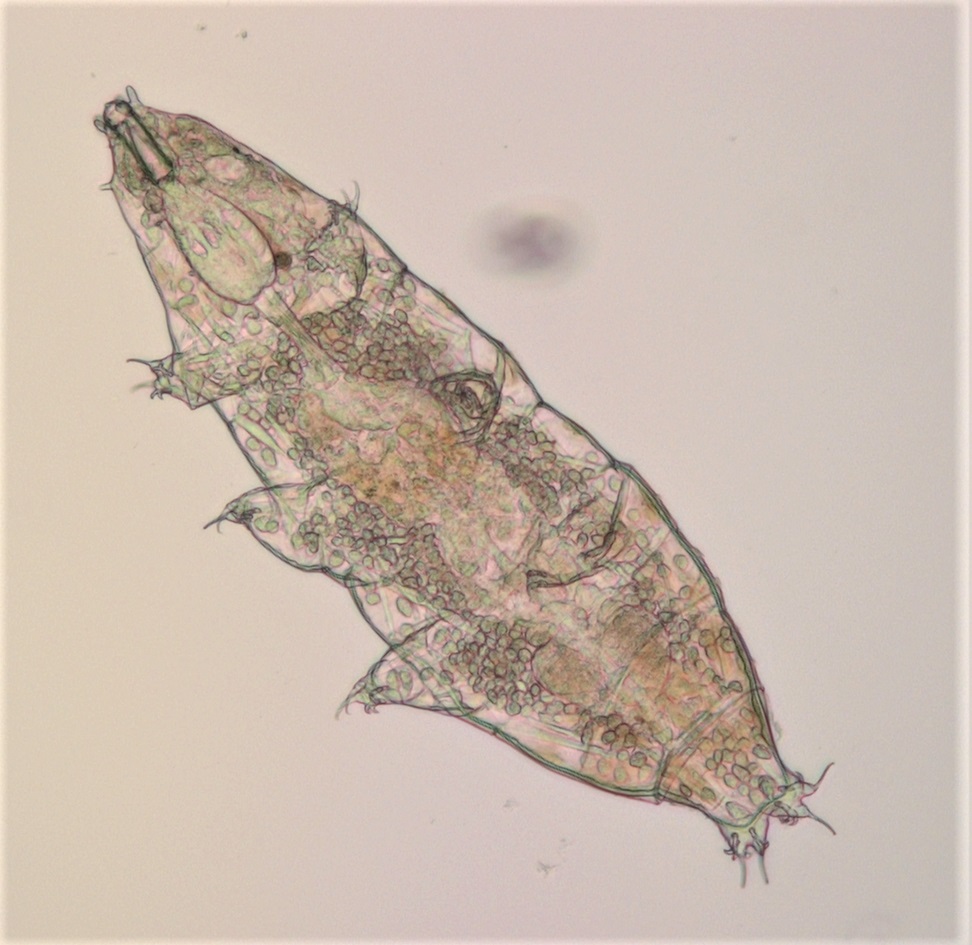
to study environmental stresses (Photo courtesy: Harikumar RS)
When I first started working with the fruit fly Drosophila melanogaster after my Master’s, many of my friends and family members were greatly amused. After 17 years of education, I had finally admitted that all I was doing was “mashya marne” or “killing flies”, a saying in Marathi that suggests wasting time. Over the years, I was often asked when I was going to “evolve” to working with more complex organisms. One engineer friend was quite disappointed that instead of designing methods to eliminate these insects from our kitchens, I was trying to understand how cells in their wings organised themselves. I patiently explained to them that most cells in our bodies need to know their top from their bottom, and their left from right. Only then could they align in specific formations and perform functions correctly. Just like soldiers on a battlefield.
“But what’s the point of studying this in flies?” they asked. “Shouldn’t you study human cells?” A fair question. But here is the point: While researchers can perform non-invasive, observation-based studies using humans, they cannot perform invasive experiments on them. Besides, human bodies are often too complex to get clear answers purely by observation. In addition, for ethical reasons, researchers cannot change or mutate humans. Finally, and most importantly, what is biologically true for flies is often true for fishes and worms, and for mice and (wo)men.
Exploiting other species
Most organisms that scientists use for asking basic biological questions are found in their immediate surroundings: flies on their fruit, mice in their basement, bacteria in their gut and yeast in their bread. These are easy to acquire and economical to raise in labs. Their life-cycle is much shorter than ours, therefore several generations can be studied in a reasonable timeframe. They often grow fast (in number), allowing statistical analysis, and they are amenable to experimentation, albeit under some ethical regulations. This has resulted in scientists developing several tools and techniques for their study. A lot of their biology can be extrapolated to other life forms. They are our models, and give us a handle to understand complex phenomena associated with life on Earth.
Humans have historically exploited other species. The pharmaceuticals industry, for example, is almost entirely built upon microbial activities. We have used them to set our curd and ferment our beer, treat our oil spills and control our pests. However, sometimes this exploitation extends beyond direct benefits. They can become our windows into fundamental life processes and help us gain a deeper understanding of nature.
When scientists study certain species in depth to ask universal biological questions, they call these species ‘model organisms’. Technologies develop around these organisms, like advanced microscopy and methods for molecular and genetic manipulation. These organisms are used to solve mysteries that may not be specific to the species to which they belong. For example, ecologists or organismal biologists may ask how honeybees foraging far away from their beehives find their way back, or how the lifestyles of birds affect the shapes of their eggs. But people using the frog Xenopus as a model organism may not necessarily be interested in the frog. They might be curious about how body axes are determined – head to tail, front to back – in the earliest stages of development, something that is easy to study in the frog where eggs are fertilised outside the mother’s body. Drosophila biologists may not care as much for the fly, as they do for how genes control traits, the tools for which are available in the flies. “Worm people” study the nematode C. elegans for its almost glass-like body, making it easy to observe the development of organs, and may not care about its symbiotic relation with fig, the fruit in which they are often found.
When scientists study certain species in depth to ask universal biological questions, they call these species ‘model organisms’
Not every model organism is useful in understanding every process. For example, one cannot study gas exchange in lungs using the fruit fly, since it has a very rudimentary respiratory system, and the development of fingers from a limb-bud cannot be studied using fish. However, there is no denying the unbelievable insights into life on Earth that scientists have gained from studying such model organisms.
Choosing the best model
For centuries, philosophers and naturalists have noticed fundamental similarities among life forms. This suggested a universality to life that was exploited by scientists, a notable example being Gregor Mendel.
Mendel, an Austrian monk and a mathematician, worked in an abbey in the mid-1800s. He was curious to know if it was possible to raise crops or breed cattle that consistently showed favourable traits in every generation. He had access to the abbey gardens and hence chose a pea plant, Pisum sativum, for his experiments. The plant grew fast, allowing him to cycle through multiple generations in one season. It had easily visible traits, and produced a large number of seeds. This was the beginning of genetics, the systematic study of inheritance.
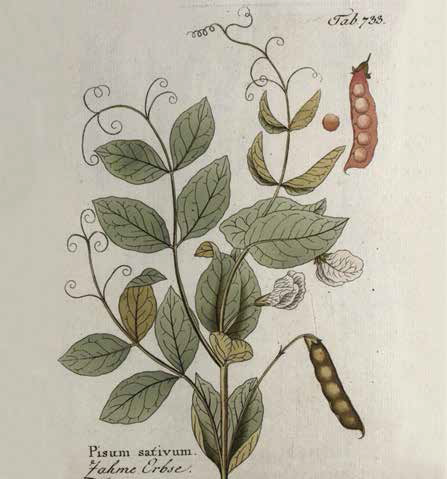
It is possible that the monk tried several other plants before zeroing in on the pea, but his choice turned out to be the right one. We know today that animals, with some exceptions, have two sets of chromosomes, the thread-like structures in the nuclei that contain the genetic material. The plant kingdom is more fluid, with some having multiple sets of chromosomes. But not the pea. It has just two copies. Mendel inadvertently chose a plant that not just gave him clear and interpretable results, but also allowed him to develop a theory that could be extrapolated to the animal world. He also realised that ‘factors’ for heritable traits (known today as genes) come in two copies, one from each parent. Any plant with more sets of chromosomes would have made Mendel’s task unenviable.
Time and again, scientists have realised that some biological mysteries are impossible to solve until the right model organism comes along. Walther Flemming and several others had noticed chromosomes in the late 1800s, in stained samples of dividing cells of the salamander. Yet, nobody connected them to Mendel’s genes, because salamander cells contained dozens of chromosomes, often tangled together. To prove that chromosomes carried hereditary information, it was important to be able to count them. It was almost 50 years after Mendel’s discovery that Theodor Boveri made a crucial observation that added a new piece to this puzzle.
Boveri was working with the roundworm (Ascaris megalocephala), which had large, transparent cells with only two pairs of chromosomes. He noticed that when germ (reproductive) cells divided to create daughter cells (sperm in males and eggs in females), each daughter cell received only one pair of chromosomes (reduction division). He also found that the sperm and egg unite to create a zygote, a fertilised egg, with two pairs of chromosomes. When the zygote divided, the two daughter cells had the same number of chromosomes as the mother cell. Boveri realised that he had uncovered the physical basis for Mendel’s laws of inheritance. He would then go on to publish beautiful drawings of equal and reduction cell divisions.
New models open new avenues
When a researcher realises that a model organism is easy to experiment on, many more researchers become drawn to it. They develop newer techniques and tools which make the study of that organism easier. Drosophila became a very attractive tool after TH Morgan, a revolutionary geneticist and embryologist in the early 1900s, and his students used it to make great strides in genetics. C. elegans became popular for those working on neural development. However, as the focus of scientists changes with new breakthroughs, the inherent limitations of established models become apparent.
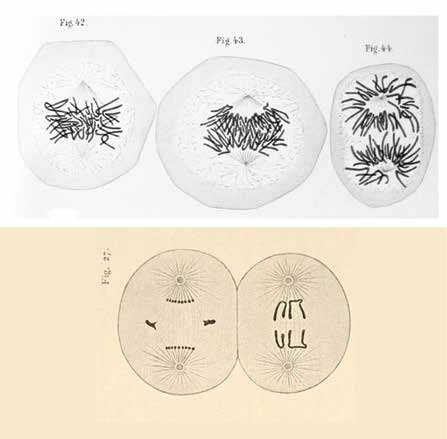
B: Illustrations of Sea urchin chromosomes by Theodor Boveri
(Images: Wikimedia Commons)
For more than a century, scientists have looked to only a handful of species to decode biological mysteries. This is restrictive, because each model organism represents only one branch of the giant evolutionary tree of life. There are numerous interesting traits and biological phenomena that are not represented in it. Therefore, scientists often search for new species, hoping to overcome old impasses or find new mysteries to solve. These new species for which scientists are yet to establish experimental techniques are the ‘non-model’ organisms.
For more than a century, scientists have looked to only a handful of species to decode biological mysteries
For example, Nipam Patel, a professor at the University of Chicago wanted to know how an organism with many pairs of appendages evolves a genetic programme where each pair is designed for a unique function. The lobster, for example, has one pair of legs for catching prey, some others for walking, some more for swimming and so on. Since working with lobsters is difficult and expensive, and because no conventional model fit the requirements, his team started looking for an alternative. One of his students found an ideal candidate, a tiny crustacean, Parhyale hawaiensis. It lived in the water filtration system in the lab, ate the garbage gathered there and was tolerant of a wide range of environmental conditions, making it quite easy to maintain in the lab. Soon, the team had identified genes that regulated the development of each appendage, found out where in the body they were active, and over a period of time, developed several experimental techniques suitable to work with this non-model species.
Model organisms at IISc
Researchers at IISc have a long history of using model and non-model organisms. The first primate centre in the country was set up here to house monkeys needed for research on reproduction. A repository of native microbes, the National Collection of Type Cultures (NCTC), was set up in what was then the Department of Fermentation Technology in 1941. Many species in the collection were used for commercial production of enzymes, fermenting sugars, and digesting different substrates to produce useful chemicals, vitamins and alcohols. The Annual Reports from 1944-45 state, “[the NCTC] has in its repository 275 bacteria, 156 fungi and 67 yeasts, making a total of 498. Microorganisms of scientific and industrial importance have been supplied to several research institutes and industrial concerns.”
In 1945-46, using the Feulgen technique, former faculty members MK Subramaniam and B Ranganathan stained the chromosomes of yeast for the first time, documenting their presence. The strain of yeast used for the study came from the NCTC. A remarkable example of the use of an unconventional organism comes from the work of PS Sarma, a professor at the Department of Biochemistry, IISc, in the 1960s. Sarma tackled the mystery of a debilitating disease, neurolathyrism, caused by eating kesari dal. This dal was cheap and was an excellent source of several nutrients, but it contained a toxin that caused muscle spasms and paralysis of lower limbs in several people who had consumed it regularly. Researchers had tried to identify the toxin by testing extracts of kesari dal on mice and rats to no avail. Sarma decided to use a grain-eating insect, Corcyra cephalonica, instead, and was able to successfully isolate the toxin, a highly acidic amino acid, and show that it had neurotoxic effects on chicken and monkeys as well.
Today, there are several faculty members at IISc who use both conventional and unconventional model organisms, such as Sandeep Eswarappa, Associate Professor in the Department of Biochemistry. Inspired by Neil Degrasse Tyson’s account of tardigrades – extremely hardy creatures that have survived five mass extinctions – he has started a project to study them in his lab. “They are not conventional model organisms, where we can manipulate their genomes or do genetic analysis,” Sandeep explains. “Tardigrades are not ready yet for genetic studies. But if you are trying to understand strategies for overcoming environmental stresses like temperature or pressure, then they are good models for that.”
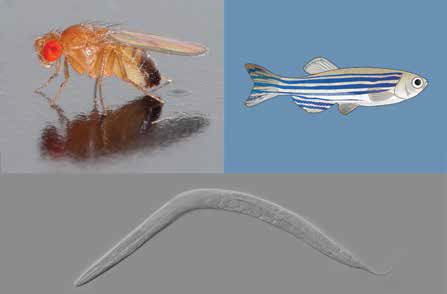
organisms (Images courtesy: Wikimedia Commons)
Another faculty member who relies on model organisms is Upendra Nongthomba, Professor at the Department of Molecular Reproduction, Development and Genetics, who studies the cellular basis of certain myopathies and neurodegenerative diseases. His lab uses two different model systems, the fruit fly Drosophila and zebrafish. Though maintaining zebrafish in a lab setting is not as easy as maintaining fruit flies, they are physiologically closer to humans. Newly developed molecular biology techniques are making it possible to use the fish for genetic studies as well. “Since our group is interested in modelling human diseases and genetic disorders, and screen drugs or formulations that alleviate the pathophysiology, the zebrafish serves as a much better model,” says Upendra. “From the disease or pathological point of view, one model can be much better than another. For example, mice never develop Duchenne Muscular Dystrophy like humans do. However, the zebrafish shows a phenotype that almost mirrors human pathology, including death at a young age.”
The search for new questions and model organisms is never ending. As Nipam Patel, in his 2019 lecture at the University of Chicago, puts it succinctly, “Biology has solved most problems. It is just a matter of finding the organism that has solved that problem, and then understanding how it has solved that problem.”