An overview of IISc labs involved in developing novel diagnostic tools for infectious diseases
With the recent emergence of the coronavirus infection, the world has been reminded of the lethal nature of disease outbreaks. Infectious diseases, caused by bacteria, viruses, protozoa, and other parasites, can be transmitted to a healthy individual from another infected individual, or via disease vectors. As a first step towards fighting any infection, diagnosis is critically important. Early detection of infected individuals helps in inhibiting further transmission. Diagnostic tests also help a physician decide whether antimicrobial treatment is necessary in a particular case or not, thus reducing the misuse of medication and the evolution of drug resistance.
India is particularly vulnerable to the spread of infectious diseases such as tuberculosis, malaria and dengue. In a tropical country like India, which is densely populated and has a large part of the population living in poverty, early diagnosis provides crucial assistance in stopping the infection from spreading and preventing a possible epidemic. However, the challenges of developing diagnostic tools are different compared to those in economically developed countries. India requires highly sensitive devices that are inexpensive, can process a large number of samples, and are easy to carry to rural areas for point-of-care tests. The development of such devices is being actively pursued in some labs at IISc.
Low-cost device for rapid testing of tuberculosis
While working as a postdoc at the University of Washington in Seattle, Bhushan Toley, now an Assistant Professor at the Department of Chemical Engineering, got introduced to paper-based microfluidic devices for biomedical diagnosis. Microfluidic devices, also known as lab-on-chip devices, process and analyse fluids in micro-channels. Paper-based materials are conventionally used in immunoassays, where the presence of a specific protein is detected by a change in colour; a famous example is the home pregnancy test. The porous nature of the paper enables fluid transport on its surface simply by wicking, eliminating the need for bulky pumps. Paper-based assays are particularly advantageous in limited-resource settings, owing to their low-cost and easy portability.
Paper-based assays are particularly advantageous in limited-resource settings, owing to their low-cost and easy portability
Early this decade, the University of Washington lab was one of the first to perform nucleic acid amplification tests (NAATs) on paper, successfully detecting bacteria that cause respiratory infections, a project in which Toley was involved. NAAT involves segregating DNA or RNA sequences of the microbe from the infected sample and amplifying them to detectable levels.
When Toley joined IISc in 2016, he wanted to use this approach to detect other infectious diseases and chose tuberculosis (TB). “TB being a major problem in India and urgently needing a rapid and inexpensive solution for diagnosis, I felt that paper-based TB diagnostics would be a very valuable solution,” he says.
Caused by Mycobacterium tuberculosis (Mtb), TB claims 1.2 million lives every year – the highest number for any single infectious disease. An estimated 10 million people were diagnosed with TB in 2018; India alone accounted for 27 percent of all TB cases detected. The Indian government launched the TB-free India campaign in early 2018, setting a target to completely eliminate the disease by 2025.
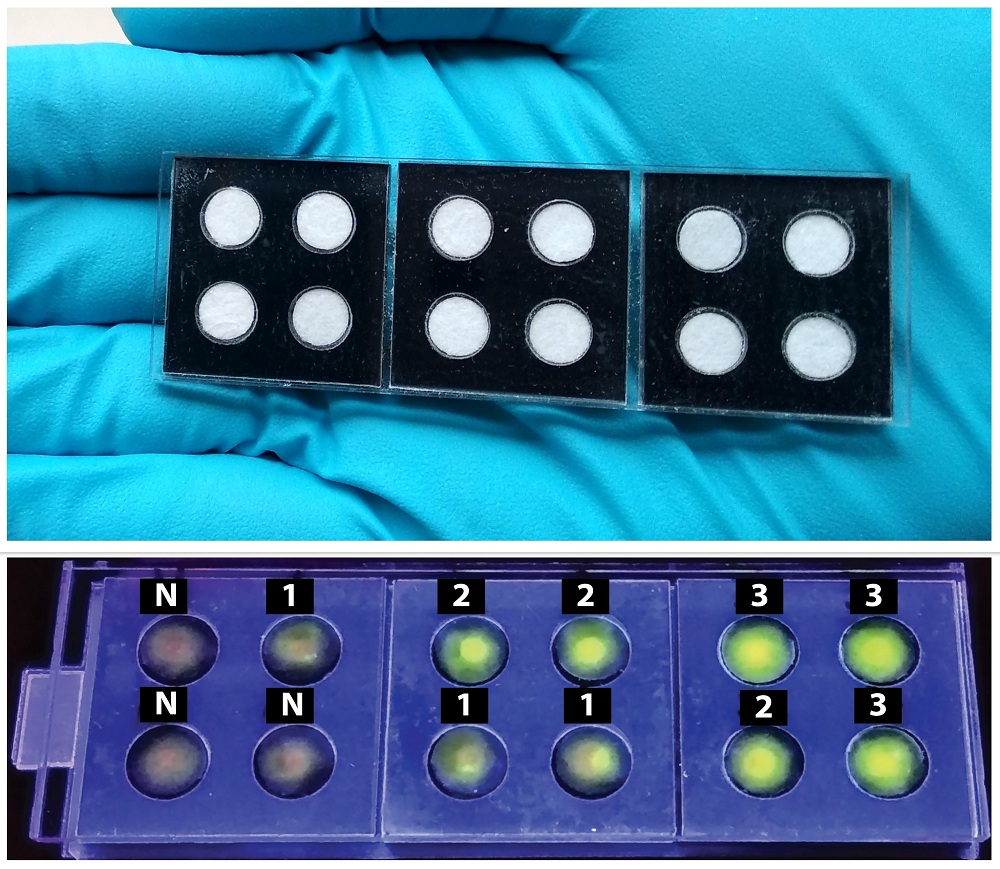
(Top) Tuberculosis diagnostic kit fabricated in Toley’s lab. Paper pads fit inside precisely cut black acrylic sheets. (Bottom) Fluorescence detection images. Numbers indicate increasing concentration of Mtb DNA (Photo: Navjot Kaur)
Toley wanted to create a low-cost diagnostic device that would work in the Indian setting. “I wanted to build a device that is uncomplicated, which even a minimally trained user can operate,” he says. Indian diagnostics labs and hospitals use smear microscopy as the first step to screen TB patients, which is not very sensitive. The current gold standard for diagnosing TB is the GeneXpert machine, which works on cartridge-based Mtb DNA amplification and is fully automated. But the machine is very expensive and a single test costs around Rs 2,000.
Along with his PhD student Navjot Kaur, Toley started developing a paper assay using inexpensive materials. They came up with a suitable design that fitted circular paper pads inside precisely cut black acrylic sheets. Purified Mtb DNA is added to the paper surface along with necessary reagents and incubated for 80 minutes at 63ºC to achieve DNA amplification. The presence of Mtb can be confirmed by shining a UV torch on the paper. “Once this device goes for commercial production, each sample test would cost less than Rs 300,” says Toley.
An analysis of clinical samples obtained from a hospital revealed 100 percent accuracy in detecting TB-positive cases. A few negative cases also showed up as positive, a problem which Toley’s group is working to resolve. They are also working on the dry storage of pathogen samples on paper for longer durations to facilitate transport of DNA samples.
‘One Health’ for animals and humans
“Our interaction with animals is changing,” says Utpal Tatu, Professor in the Department of Biochemistry. “We share not only the environment but also the diseases,” he adds, emphasising the need to keep animals healthy. Many emerging infectious diseases – SARS, bird flu, Ebola, and possibly COVID-19 – originated in animals.
Tatu joined IISc in 1997 and has been involved in studying the proteomics of pathogens causing neglected infectious diseases such as malaria, amoebiasis, and giardiasis. Proteomics, or identifying and analysing proteins produced by an organism, is beneficial in developing drugs that target and disable key proteins, inhibiting growth and virulence. It also helps identify specific protein antigens produced by the pathogen that can be used as biomarkers for diagnosis. “Among all the diseases studied, our laboratory has achieved significant progress in bringing two potential diagnostic studies to fruition in the form of kits: for trypanosome infection in animals, and for trichomonas infection in humans,” he says.
Tatu’s association with animal diseases started when one of his friends asked him to take a look at his sick horse. It was unclear what the horse suffered from; after vigorously nodding its head due to pain for a day, the horse died the next day. Over time, with more animals across India succumbing to this unknown sickness, Tatu was approached by the animal husbandry department to help identify it. Analysing blood samples collected from sick cattle, he found that they were infected with a protozoan called Trypanosoma evansi, a parasite which had eluded detection. Once the cause was determined, the cattle could be treated with medication.
In India, trypanosome infection predominantly affects cattle, resulting in a significant loss of livestock and is a huge economic burden on farmers. Tatu collected animal blood samples from across India and analysed the proteins of the infecting microbe. Eventually, antigen-based rapid detection kits were fabricated to detect T. evansi presence. “The kit, which relies on a drop of blood, can diagnose if the animal is infected or not within ten minutes,” he says. Tatu’s lab is currently supplying these low-cost kits to many veterinarians and turf clubs. In the near future, with funding from the Biotechnology Industry Research Assistance Council (BIRAC), Tatu aims to reach farmers directly by scaling up marketing.
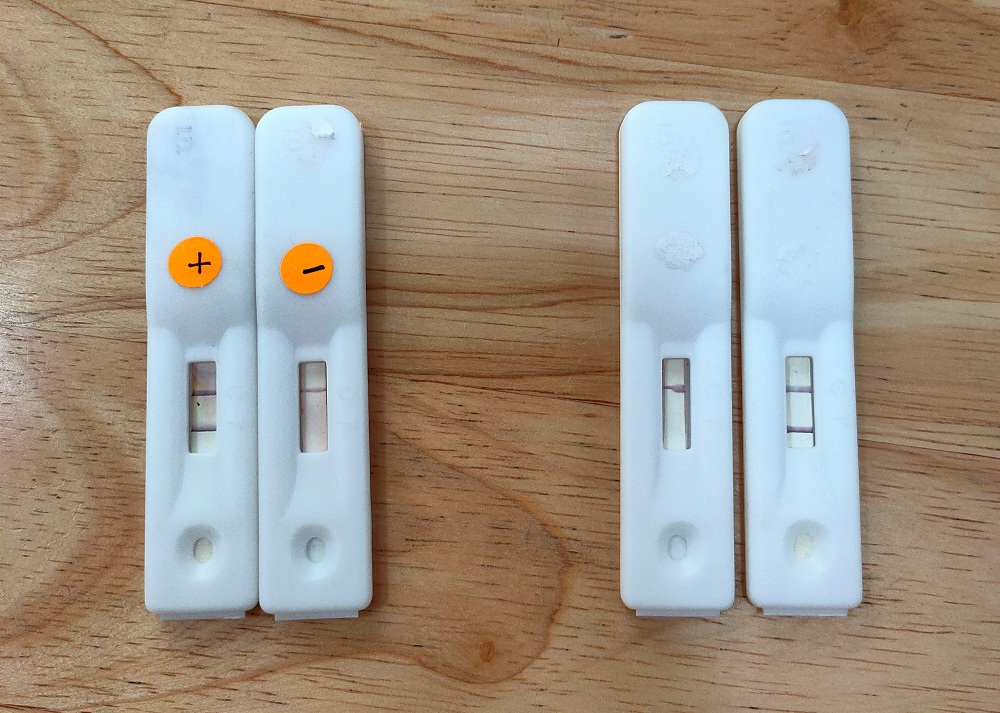
Antigen-based rapid diagnostic kits fabricated in Tatu’s lab for trypanosomiasis (left) and trichomoniasis (right). Two coloured lines in the assay indicate the presence of infection (Photo: Neelima Basavaraju)
Tatu’s lab has also developed a diagnostic kit for trichomoniasis, a sexually transmitted disease (STD) caused by another protozoan Trichomonas vaginalis, which infects the human genitourinary tract. This infection mainly affects women. However, there were no effective detection methods for this disease. Collaborating with gynaecologists in Bangalore, Tatu obtained infected genital secretion samples to analyse the proteins of the pathogen. He successfully identified the biomarker needed to diagnose this disease. These kits, after successful clinical evaluations, are currently being used by clinicians in Bangalore.
Malaria diagnosis by trapping red blood cells
Vasant Natarajan, Professor in the Department of Physics, joined IISc in 1996 after working at AT&T Bell Labs, New Jersey. “[I wanted] to do research relevant to the needs of Indian society, and not the kind of esoteric research that happens at Bell Labs,” he says. At IISc, he chose to work on malaria.
Malaria is caused by single-celled Plasmodium parasites, and transmitted by the bite of an infected female Anopheles mosquito. Globally, 228 million people were diagnosed with malaria in 2018, almost all of them in tropical and subtropical countries. In 2017, India recorded 8.8 million malaria cases. To detect malaria infection, two methods are widely employed: blood smear microscopy and antigen-based rapid diagnostic tests.
“Natarajan came up with a totally new idea for diagnosing malaria. Up until now, biologists were interested in looking at microscopes and developing biomarkers in a conventional way,” says Tatu, who is one of the collaborators on this project. “Natarajan decided to explore the change in physical properties of the infected human cells.” Malarial parasites enter red blood cells (RBCs) and multiply, changing the shape and size of infected RBCs. The parasites also secrete numerous proteins to help them survive, resulting in increased rigidity of the RBC membrane and increased adhesion of RBCs to vascular surfaces.
“Natarajan came up with a totally new idea for diagnosing malaria,” says Tatu
Natarajan probed these changes by trapping the blood cells optically, using focused laser beams to apply force and physically hold a single RBC, similar to mechanical tweezers. “The idea was to see whether the malaria infection changes the corner frequency of the RBC,” says Apurba Paul, a former PhD student who worked on this project and is currently a postdoc at Clemson University, South Carolina. Corner frequency gives a measure of the stiffness of the trapped molecule and varies based on the change in the molecule’s physical properties.
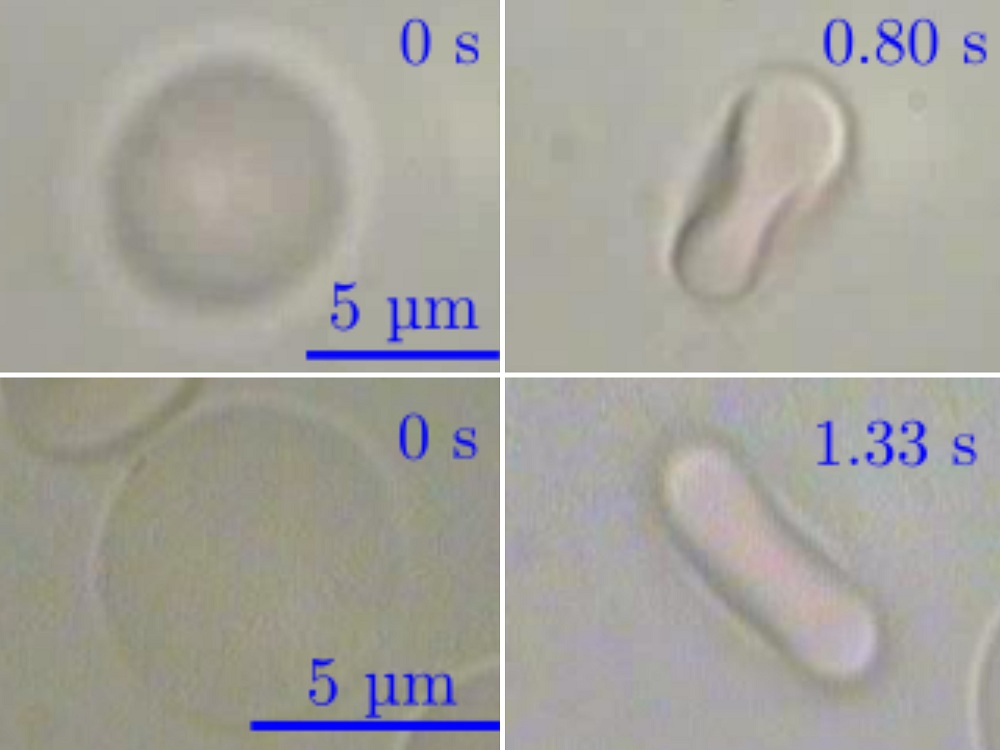
RBC from a healthy person (top) vs Plasmodium-infected RBC (bottom). When optically trapped (right), the cells fold. Time taken to completely fold is marked on their pictures (Image: Apurba Paul)
Natarajan’s team found that the corner frequency noticeably increased for infected RBCs, compared to healthy ones. They also found that infected RBCs showed an increase in corner frequency even if it didn’t contain the parasite, a finding that they termed as ‘bystander effect’. “This effect may be caused by the substances secreted into the bloodstream by the parasite or the infected RBCs,” explains Paul. “We have tried to isolate these substances, and the results suggest that cyclic adenosine monophosphate (cAMP) could be one of them,” he adds. This effect enables the detection of malaria earlier than existing methods, even when the parasite count in blood is very low. The group has validated this technique on clinical samples obtained from malaria-infected patients.
Point-of-care diagnostics: taking the lab to the field
To diagnose malaria, blood smear microscopy is the most widely used method. But its reliability depends largely on the proficiency of the technician performing it, especially when the pathogen count in the blood is low. Accurately detecting infection through microscopy takes a few hours. A wholly automated machine used for complete analysis is often bulky and expensive.
“For malaria diagnosis, we have picked up the gold standard of microscopy, and we are trying to translate it into a point-of-care device by bringing in end-to-end automation,” says Sai Siva Gorthi, Assistant Professor at the Department of Instrumentation and Applied Physics. Gorthi’s lab has developed an automated device consisting of a single unit with a microscope, sample holder, optical setup and computational tools, all inside a compact box, making it easily portable. In an actual clinical setting, the technician would just have to mix the blood sample with the reagents, drop the fluid onto the provided microfluidic slide, and keep it inside the device.
“The end-to-end automation of our device avoids the requirement of a skilled technician,” points out Gorthi. The machine uses algorithms to focus on infected RBCs, acquire images, and present the result. “The result can be known within ten minutes,” says Gorthi. The device is equipped to check for both the life-stage of Plasmodium as well as the parasite count. It can also be used to detect an array of other RBC-related disorders, such as sickle cell anaemia and spherocytosis, and can easily be extended for routine tests of blood cell counts.
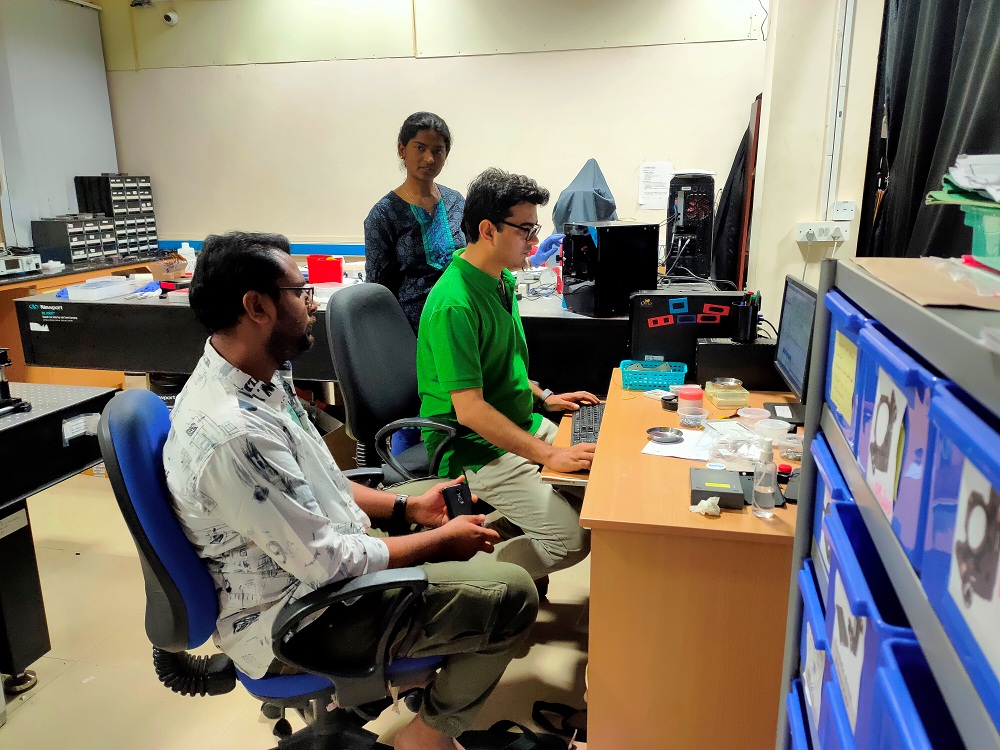
Students from Gorthi’s lab (Aravind Venukumar, Prateek Katare, and Roopa Ashwath) loading samples on their lab-built device and analysing the images (Photo: Neelima Basavaraju)
After clinical validation supported by BIRAC, Gorthi aims to commercialise the device through a startup incubated at the Society for Innovation and Development, IISc. He is also working with the Indian Council of Medical Research to take the device to North East India for large-scale malaria detection.
Neelima Basavaraju, a former postdoctoral researcher at IISc’s Solid State and Structural Chemistry Unit, is a freelance writer.